Difference between revisions of "Evidence of evolution" - New World Encyclopedia
Rick Swarts (talk | contribs) |
Rick Swarts (talk | contribs) |
||
Line 14: | Line 14: | ||
refers to changes in gene frequencies ..... | refers to changes in gene frequencies ..... | ||
+ | Notes: | ||
+ | Broadly defined, '''evolution''' is any heritable change in a population of organisms over time. Changes may be slight or large, but must be passed on to the next generation (or many generations) and must involve populations, not individuals. A popular definition along these lines is that offered by Douglas J. Futuyama (1986) in ''Evolutionary Biology:'' "Biological evolution…is change in the properties of populations of organisms that transcend the lifetime of a single individual…. The changes in populations that are considered evolutionary are those that are inheritable via the genetic material from one generation to another." | ||
+ | |||
+ | Similarly, the term may be presented in terms of [[allele]] frequency (with an "allele" being an alternative form of a gene, such as different alleles code for different eye colors): "Evolution can be precisely defined as any change in the frequency of alleles within a gene pool from one generation to the next" (Curtis & Barnes, 1989). | ||
+ | Both a slight change (as in pesticide resistance in a strain of bacteria) and a large change (as in the development of major new designs such as feathered wings for flying, or even the present diversity of life from simple prokaryotes) qualify as evolution. | ||
+ | However, the term evolution is often used with more narrow meanings. It is not uncommon to see the term equated to the specific theory that all organisms have descended from common ancestors (which is also known as the "theory of descent with modification"). Less frequently, evolution is sometimes used to refer to one explanation for the process by which change occurs, the "theory of modification through [[natural selection]]." In addition, the term evolution is occasionally used with reference to a comprehensive theory that includes both the non-causal pattern of descent with modification and the causal mechanism of natural selection. | ||
+ | |||
+ | As broadly and commonly defined in the scientific community, the term evolution connotes heritable changes in populations of organisms over time, or changes in the frequencies of alleles over time. In this sense, the term does not specify any overall pattern of change through the ages, nor the process whereby change occurs (although the term is also employed in such a manner). | ||
+ | |||
+ | However, there are two very important and popular evolutionary theories that address the pattern and process of evolution: "theory of descent with modification" and "theory of natural selection," respectively, as well as other concepts in evolutionary theory that deal with speciation and the rate of evolution. | ||
+ | |||
+ | Theory of descent with modification | ||
+ | |||
+ | The "theory of descent with modification" is the major kinematic theory that deals with the pattern of evolution—that is, it treats non-causal relations between ancestral and descendent [[species]], orders, phyla, and so forth. The theory of descent with modification, also called the "theory of common descent," essentially postulates that all organisms have descended from common ancestors by a continuous process of branching. In other words, all life evolved from one kind of organism or from a few simple kinds, and each species arose in a single geographic location from another species that preceded it in time. Each group of organisms shares a common ancestor. | ||
+ | |||
+ | One of the major contributions of [[Charles Darwin]] was to marshal substantial evidence for the theory of descent with modification, particularly in his book, ''Origin of Species.'' Among the evidences that evolutionists use to document the "pattern of evolution" are the fossil record, the distribution patterns of existing species, methods of dating fossils, and comparison of homologous structures. (See [[Evolution#Evidences_of_Evolution|Evidences of Evolution]] below.) | ||
+ | |||
+ | Theory of natural selection | ||
+ | |||
+ | ''Main articles:'' [[Darwinism]] and [[Natural selection]] | ||
+ | |||
+ | The second major evolutionary theory is the "theory of modification through natural selection," also known as the "theory of natural selection." This is a dynamic theory that involves mechanisms and causal relationships. The theory of natural selection is one explanation offered for how evolution might have occurred; in other words, the "process" by which evolution took place to arrive at the pattern. | ||
+ | |||
+ | Natural selection may be defined as the mechanism whereby biological individuals that are endowed with favorable or deleterious traits reproduce more or less than other individuals that do not possess such traits. | ||
+ | |||
+ | According to this theory, natural selection is the directing or creative force of evolution. | ||
Note: This is only a very rough draft, with notes that may be useful in developing the article. Please do not edit this article until the actual article is complete — i.e., when this notice is removed. You may add comments on what you would like to see included. [[User:Rick Swarts|Rick Swarts]] 00:05, 28 Sep 2005 (UTC) | Note: This is only a very rough draft, with notes that may be useful in developing the article. Please do not edit this article until the actual article is complete — i.e., when this notice is removed. You may add comments on what you would like to see included. [[User:Rick Swarts|Rick Swarts]] 00:05, 28 Sep 2005 (UTC) | ||
Line 36: | Line 62: | ||
+ | [[Image:HMSBeagle.jpeg|thumb|right|300px|While on board ''[[HMS Beagle]]'', [[Charles Darwin]] collected numerous specimens, many new to science, which supported his later theory of evolution by [[natural selection]].]] | ||
+ | The wide range of '''evidence of [[evolution]]''' provides a wealth of information on the natural processes by which the variety of life on Earth developed. | ||
− | [[ | + | [[Fossil]]s are important for estimating when various lineages developed. As fossilization is an uncommon occurrence, usually requiring hard body parts and death near a site where [[sediment]]s are being deposited, the [[fossil record]] only provides sparse and intermittent information about the [[evolution]] of life. [[scientific evidence|Evidence]] of organisms prior to the development of hard body parts such as shells, bones and teeth is especially scarce, but exists in the form of ancient [[microfossil]]s, as well as impressions of various soft-bodied organisms. |
− | + | Comparison of the genetic sequence of organisms has revealed that organisms that are [[phylogeny|phylogenetically]] close have a higher degree of sequence similarity than organisms that are phylogenetically distant. Further evidence for [[common descent]] comes from genetic detritus such as [[pseudogene]]s, regions of DNA that are [[ortholog]]ous to a gene in a related organism, but are no longer active and appear to be undergoing a steady process of degeneration. Since [[metabolism|metabolic]] processes do not leave fossils, research into the evolution of the basic cellular processes is also done largely by comparison of existing organisms. Many lineages diverged at different stages of development, so it is theoretically possible to determine when certain metabolic processes appeared by comparing the traits of the descendants of a common ancestor. | |
− | |||
− | + | ==Evidence from paleontology== | |
+ | [[Image:Resin with insect (aka).jpg|thumb|200px|right|An insect trapped in [[amber]].]] | ||
− | + | When organisms die, they often [[decomposition|decompose]] rapidly or are consumed by [[scavenger]]s, leaving no permanent evidences of their existence. However, occasionally, some organisms are preserved. The remains or traces of organisms from a past [[geologic time scale|geologic age]] embedded in [[rock (geology)|rocks]] by natural processes are called [[fossil]]s. They are extremely important for understanding the [[timeline of evolution|evolutionary history of life]] on Earth, as they provide direct evidence of evolution and detailed information on the ancestry of organisms. [[Paleontology]] is the study of past life based on fossil records and their relations to different geologic time periods. | |
− | + | For fossilization to take place, the traces and remains of organisms must be quickly buried so that weathering and decomposition do not occur. Skeletal structures or other hard parts of the organisms are the most commonly occurring form of fossilized remains (Paul, 1998), (Behrensmeyer, 1980) and (Martin, 1999). There are also some trace "fossils" showing [[molding (process)|moulds]], cast or imprints of some previous organisms. | |
− | + | As an animal dies, the organic materials gradually decay, such that the [[bone]]s become porous. If the animal is subsequently buried in [[mud]], [[mineral]] salts will infiltrate into the bones and gradually fill up the pores. The bones will harden into stones and be preserved as fossils. This process is known as [[petrification]]. If dead animals are covered by wind-blown [[sand]], and if the sand is subsequently turned into mud by heavy [[rain]] or [[flood]]s, the same process of mineral infiltration may occur. Apart from petrification, the dead bodies of organisms may be well preserved in [[ice]], in hardened [[resin]] of [[pinophyta|coniferous]] trees ([[amber]]), in tar, or in anaerobic, [[acid]]ic [[peat]]. Fossilization can sometimes be a [[trace]], an impression of a form. Examples include leaves and footprints, the fossils of which are made in layers that then harden. | |
− | [[ | ||
− | + | ===Fossil records=== | |
+ | {{main|Fossil record}} | ||
− | + | [[Image:Asaphiscuswheelerii.jpg|thumb|200px|left|Fossil [[trilobite]]. Trilobites were hard-shelled arthropods, related to living [[horseshoe crab]]s and [[spider]]s, that first appeared in significant numbers around 540 [[mya (unit)|mya]], [[extinction|dying out]] 250 mya.]] | |
− | + | It is possible to find out how a particular group of organisms evolved by arranging its fossil records in a chronological sequence. Such a sequence can be determined because fossils are mainly found in [[sedimentary rock]]. Sedimentary rock is formed by layers of [[silt]] or mud on top of each other; thus, the resulting rock contains a series of horizontal layers, or [[stratum|strata]]. Each layer contains fossils which are typical for a specific [[period (geology)|time period]] during which they were made. The lowest strata contain the oldest rock and the earliest fossils, while the highest strata contain the youngest rock and more recent fossils. | |
− | + | A [[succession]] of animals and plants can also be seen from fossil records. Fossil evidence supports the theory that organisms tend to progressively increase in complexity. By studying the number and complexity of different fossils at different [[stratigraphy|stratigraphic]] levels, it has been shown that older fossil-bearing rocks contain fewer types of fossilized organisms, and they all have a simpler structure, whereas younger rocks contain a greater variety of fossils, often with increasingly complex structures. | |
− | [[ | + | |
+ | In the past, geologists could only roughly estimate the ages of various strata and the fossils found. They did so, for instance, by estimating the time for the formation of sedimentary rock layer by layer. Today, by measuring the proportions of [[radioactive decay|radioactive]] and stable [[chemical element|elements]] in a given rock, the ages of fossils can be more precisely dated by scientists. This technique is known as [[radiometric dating]]. | ||
− | + | Throughout the fossil record, many species that appear at an early stratigraphic level disappear at a later level. This is interpreted in evolutionary terms as indicating the times at which species originated and became extinct. Geographical regions and climatic conditions have varied throughout the Earth's history. Since organisms are adapted to particular environments, the constantly changing conditions favoured species which adapted to new environments through the mechanism of [[natural selection]]. | |
− | + | According to fossil records, some modern species of plants and animals are found to be almost identical to the species that lived in ancient geological ages. They are existing species of ancient lineages that have remained [[morphology (biology)|morphologically]] (and probably also [[physiology|physiologically]]) somewhat unchanged for a very long time. Consequently, they are called "[[living fossil]]s" by laypeople. Examples of "living fossils" include the [[tuatara]], the [[nautilus]], the [[horseshoe crab]], the [[coelacanth]], the [[ginkgo]], the [[Wollemi pine]], and the [[metasequoia]]. | |
− | |||
− | |||
− | + | ====Extent of the Fossil Record==== | |
+ | Despite the relative rarity of suitable conditions for fossilization, approximately 250,000 fossil species are known[http://www.gpc.edu/~pgore/geology/historical_lab/preservationlab.php]. The number of individual fossils this represents varies greatly from species to species, but many millions of fossils have been recovered: for instance, more than three million fossils from the last [[Ice Age]] have been recovered from the [[La Brea Tar Pits]] in Los Angeles[http://www.tarpits.org/education/guide/index.html]. Many more fossils are still in the ground, in various geological formations known to contain a high fossil density, allowing estimates of the total fossil content of the formation to be made. An example of this occurs in South Africa's Beaufort Formation (part of the Karoo Supergroup, which covers most of South Africa), which is rich in vertebrate fossils, including [[therapsids]] (reptile/mammal [[Transitional fossil|transitional forms]])[http://www.palaeos.com/Paleozoic/Permian/Karroo.htm]. It has been estimated[http://www.lhup.edu/~dsimanek/6flood.htm] that this formation contains 800 billion vertebrate fossils. | ||
− | === | + | ===Evolution of the horse=== |
− | [[Image:Horseevolution.png| | + | :''Further information: [[Evolution of the horse]]'' |
+ | [[Image:Horseevolution.png|275px|thumb|right|[[Evolution of the horse]] showing reconstruction of the fossil species obtained from successive rock strata. The foot diagrams are all front views of the left forefoot. The third [[metacarpal]] is shaded throughout. The teeth are shown in longitudinal section.]] | ||
− | + | Due to an almost-complete fossil record found in [[North America]]n sedimentary deposits from the early [[Eocene]] to the present, the [[horse]] provides one of the best examples of evolutionary history ([[phylogeny]]). | |
− | + | This evolutionary sequence starts with a small animal called the ''[[Hyracotherium]]'' which lived in North America about 54 million years ago, then spread across to [[Europe]] and [[Asia]]. Fossil remains of ''Hyracotherium'' show it to have differed from the modern horse in three important respects: it was a small animal (the size of a [[fox]]), lightly built and adapted for running; the limbs were short and slender, and the feet elongated so that the digits were almost vertical, with four digits in the [[forelimb]]s and three digits in the [[hindlimb]]s; and the [[incisor]]s were small, the [[Molar (tooth)|molar]]s having low crowns with rounded [[cusp]]s covered in [[Tooth enamel|enamel]]. | |
− | |||
− | |||
− | |||
− | The probable course of development of horses from | + | The probable course of development of horses from ''Hyracotherium'' to ''Equus'' (the modern horse) involved at least 12 [[genus|genera]] and several hundred [[species]]. The major trends seen in the development of the horse to changing environmental conditions may be summarized as follows: |
− | * | + | * Increase in size (from 0.4 m to 1.5 m); |
− | * | + | * Lengthening of limbs and feet; |
− | * | + | * Reduction of lateral digits; |
− | * | + | * Increase in length and thickness of the third digit; |
− | * | + | * Increase in width of [[incisor]]s; |
− | * | + | * Replacement of [[premolar]]s by [[Molar (tooth)|molar]]s; and |
− | * | + | * Increases in tooth length, crown height of molars. |
− | + | Fossilized plants found in different strata show that the [[marsh]]y, wooded country in which ''Hyracotherium'' lived became gradually drier. Survival now depended on the head being in an elevated position for gaining a good view of the surrounding countryside, and on a high turn of speed for escape from [[predation|predators]], hence the increase in size and the replacement of the splayed-out foot by the hoofed foot. The drier, harder ground would make the original splayed-out foot unnecessary for support. The changes in the teeth can be explained by assuming that the diet changed from soft [[vegetation]] to [[grass]]. A dominant genus from each geological period has been selected to show the progressive development of the horse. However, it is important to note that there is no evidence that the forms illustrated are direct descendants of each other, even though they are related. | |
===Limitations=== | ===Limitations=== | ||
− | + | The fossil record is an important source for scientists when tracing the evolutionary history of organisms. However, because of limitations inherent in the record, there are not fine scales of intermediate forms between related groups of species. This lack of continuous fossils in the record is a major limitation in tracing the descent of biological groups. Furthermore, there are also much larger gaps between major evolutionary lineages. These gaps are often referred to as "[[transitional fossil|missing links]]". | |
− | The | + | There is a gap of about 100 million years between the early [[Cambrian]] period and the later [[Ordovician]] period. The early Cambrian period was the period from which numerous fossils of [[sea sponge|sponge]]s, [[cnidarian]]s (''e.g.'', [[jellyfish]]), [[echinoderm]]s (''e.g.'', [[eocrinoid]]s), [[molluscs]] (''e.g.'', [[snail]]s) and [[arthropod]]s (''e.g.'', [[trilobite]]s) are found. In the later Ordovician period, the first animal that really possessed the typical features of [[vertebrates]], the [[Australia]]n [[fish]], ''[[Arandaspis]]'' appeared. Thus few, if any, fossils of an intermediate type between [[invertebrate]]s and vertebrates have been found, although likely candidates include the [[Burgess Shale]] animal, ''[[Pikaia gracilens]]'', and its [[Maotianshan Shales]] relatives, ''[[Myllokunmingia]]'', ''[[Yunnanozoon]]'', ''[[Haikouella lanceolata]]'', and ''[[Haikouichthys]]''. |
− | + | Some of the reasons for the incompleteness of fossil records are: | |
− | + | * In general, the probability that an organism becomes fossilized after death is very low; | |
− | + | * Some species or groups are less likely to become fossils because they are soft-bodied; | |
− | * In general, the | + | * Some species or groups are less likely to become fossils because they live (and die) in conditions that are not favourable for fossilization to occur in; |
− | * | + | * Many fossils have been destroyed through erosion and tectonic movements; |
− | * | + | * Some fossil remains are complete, but most are fragmentary; |
− | * | + | * Some evolutionary change occurs in populations at the limits of a species' ecological range, and as these populations are likely to be small, the probability of fossilization is lower (see [[punctuated equilibrium]]); |
− | + | * Similarly, when environmental conditions change, the population of a species is likely to be greatly reduced, such that any evolutionary change induced by these new conditions is less likely to be fossilized; | |
− | * | + | * Most fossils convey information about external form, but little about how the organism functioned; |
− | + | * Using present-day biodiversity as a guide, this suggests that the fossils unearthed represent only a small fraction of the large number of species of organisms that lived in the past. | |
− | |||
− | |||
− | |||
==Evidence from comparative anatomy== | ==Evidence from comparative anatomy== | ||
− | [[Comparative anatomy|Comparative study of the anatomy]] of groups of animals or plants reveals that certain structural features are basically similar. For example, the basic structure of all [[flower]]s consists of [[sepal]]s, [[petal]]s, [[stigma | + | [[Comparative anatomy|Comparative study of the anatomy]] of groups of animals or plants reveals that certain structural features are basically similar. For example, the basic structure of all [[flower]]s consists of [[sepal]]s, [[petal]]s, [[carpel|stigma, style and ovary]]; yet the [[size]], [[colour]], [[number]] of parts and specific structure are different for each individual species. |
===Homologous structures and divergent (adaptive) evolution=== | ===Homologous structures and divergent (adaptive) evolution=== | ||
If widely separated groups of organisms are originated from a common ancestry, they are expected to have certain basic features in common. The degree of [[similarity|resemblance]] between two organisms should indicate how closely related they are in evolution: | If widely separated groups of organisms are originated from a common ancestry, they are expected to have certain basic features in common. The degree of [[similarity|resemblance]] between two organisms should indicate how closely related they are in evolution: | ||
* Groups with little in common are assumed to have diverged from a [[common ancestor]] much earlier in geological history than groups which have a lot in common; | * Groups with little in common are assumed to have diverged from a [[common ancestor]] much earlier in geological history than groups which have a lot in common; | ||
− | * | + | * In deciding how closely related two animals are, a comparative anatomist looks for [[structure]]s that are fundamentally similar, even though they may serve different functions in the [[adult]]. Such structures are described as [[Homology (biology)|homologous]] and suggest a common origin. |
− | * | + | * In cases where the similar structures serve different functions in adults, it may be necessary to trace their origin and embryonic development. A similar developmental origin suggests they are the same structure, and thus likely to be derived from a common ancestor. |
− | When a group of | + | When a group of organisms share a homologous structure which is specialized to perform a variety of functions in order to adapt different environmental conditions and modes of life are called [[adaptive radiation]]. The gradual spreading of organisms with adaptive radiation is known as [[divergent evolution]]. |
====Pentadactyl limb==== | ====Pentadactyl limb==== | ||
− | [[Image:Evolution pl.png|thumb|right|500px|'''Figure 5a''': The principle of [[homology]] illustrated by the adaptive radiation of the forelimb of mammals. All conform to the basic pentadactyl pattern but are modified for different usages. The third metacarpal is shaded throughout; the shoulder is crossed-hatched.]] | + | [[Image:Evolution pl.png|thumb|right|500px|'''Figure 5a''': The principle of [[homology (biology)|homology]] illustrated by the adaptive radiation of the forelimb of mammals. All conform to the basic pentadactyl pattern but are modified for different usages. The third metacarpal is shaded throughout; the shoulder is crossed-hatched.]] |
− | The pattern of limb bones called [[pentadactyl limb]] is an example of homologous structures (Fig. 5a). It is found in all classes of [[tetrapod]]s (i.e. from [[amphibian]]s to [[mammal]]s). It can even be traced back to the [[fin]]s of certain fossil fishes from which the first amphibians are thought to have evolved. The limb has a single proximal bone ([[humerus]]), two distal bones ([[radius]] and [[ulna]]), a series of [[carpal]]s ([[wrist]] bones), followed by five series of metacarpals ([[palm]] bones) and [[phalange]]s (digits). Throughout the tetrapods, the fundamental structures of pentadactyl limbs are the same, indicating that they originated from a common ancestor. But in the course of evolution, these fundamental structures have been modified. They have become superficially different and unrelated structures to serve different functions in | + | The pattern of limb bones called [[pentadactyl limb]] is an example of homologous structures (Fig. 5a). It is found in all classes of [[tetrapod]]s (''i.e.'' from [[amphibian]]s to [[mammal]]s). It can even be traced back to the [[fin]]s of certain fossil fishes from which the first amphibians are thought to have evolved. The limb has a single proximal bone ([[humerus]]), two distal bones ([[radius]] and [[ulna]]), a series of [[carpal]]s ([[wrist]] bones), followed by five series of metacarpals ([[hand|palm]] bones) and [[phalange]]s (digits). Throughout the tetrapods, the fundamental structures of pentadactyl limbs are the same, indicating that they originated from a common ancestor. But in the course of evolution, these fundamental structures have been modified. They have become superficially different and unrelated structures to serve different functions in adaptation to different environments and modes of life. This phenomenon is clearly shown in the forelimbs of mammals. For example: |
* In the [[monkey]], the forelimbs are much elongated to form a grasping hand for climbing and swinging among trees. | * In the [[monkey]], the forelimbs are much elongated to form a grasping hand for climbing and swinging among trees. | ||
* In the [[pig]], the first digit is lost, and the second and fifth digits are reduced. The remaining two digits are longer and stouter than the rest and bear a hoof for supporting the body. | * In the [[pig]], the first digit is lost, and the second and fifth digits are reduced. The remaining two digits are longer and stouter than the rest and bear a hoof for supporting the body. | ||
* In the horse, the forelimbs are adapted for support and running by great elongation of the third digit bearing a hoof. | * In the horse, the forelimbs are adapted for support and running by great elongation of the third digit bearing a hoof. | ||
− | * The [[mole]] has a pair of short, spade-like forelimbs for [[burrowing]]. | + | * The [[mole (animal)|mole]] has a pair of short, spade-like forelimbs for [[burrowing]]. |
* The [[anteater]] uses its enlarged third digit for tearing down [[ant]] hills and [[termite]] nests. | * The [[anteater]] uses its enlarged third digit for tearing down [[ant]] hills and [[termite]] nests. | ||
* In the [[whale]], the forelimbs become [[flipper]]s for steering and maintaining equilibrium during swimming. | * In the [[whale]], the forelimbs become [[flipper]]s for steering and maintaining equilibrium during swimming. | ||
* In the [[bat]], the forelimbs have turned into [[wing]]s for flying by great elongation of four digits, and the [[hook]]-like first digit remains free for hanging from [[tree]]s. | * In the [[bat]], the forelimbs have turned into [[wing]]s for flying by great elongation of four digits, and the [[hook]]-like first digit remains free for hanging from [[tree]]s. | ||
− | ====Insect | + | ====Insect mouthparts==== |
− | [[Image:Evolution insect mouthparts.png|thumb|left|250px|'''Figure 5b''': [[Adaptive radiation]] of insect mouthparts: a, [[antenna]] | + | [[Image:Evolution insect mouthparts.png|thumb|left|250px|'''Figure 5b''': [[Adaptive radiation]] of insect mouthparts: a, [[antenna (biology)|antennae]]; c, [[compound eye]]; lb, labrium; lr, labrum; md, mandibles; mx, maxillae.]] |
− | The basic structures are the same which include a [[labrum]] (upper lip), a pair of [[mandible]]s, a [[hypopharynx]] ( | + | The basic structures are the same which include a [[labrum]] (upper lip), a pair of [[mandible]]s, a [[hypopharynx]] (floor of mouth), a pair of [[maxillae]] and a [[labium]]. These structures are enlarged and modified; others are reduced and lost. The modifications enable the insects to exploit a variety of food materials (Fig. 5b): |
− | (A) Primitive state — biting and chewing: e.g. [[grasshopper]]. Strong mandibles and maxillae for manipulating food. | + | (A) Primitive state — biting and chewing: ''e.g.'' [[grasshopper]]. Strong mandibles and maxillae for manipulating food. |
− | (B) Ticking and biting: e.g. [[honey bee]]. Labium long to lap up [[nectar]]; mandibles chew [[pollen]] and mould [[wax]]. | + | (B) Ticking and biting: ''e.g.'' [[honey bee]]. Labium long to lap up [[nectar]]; mandibles chew [[pollen]] and mould [[wax]]. |
− | (C) Sucking: e.g. [[butterfly]]. Labrum reduced; mandibles lost; maxillae long forming sucking tube | + | (C) Sucking: ''e.g.'' [[butterfly]]. Labrum reduced; mandibles lost; maxillae long forming sucking tube. |
− | (D) Piercing and sucking | + | (D) Piercing and sucking, ''e.g''. [[mosquito|female mosquito]]. Labrum and maxillae form tube; mandibles form piercing stylets; labrum grooved to hold other parts. |
+ | |||
+ | ====Other Arthropod Appendages ==== | ||
+ | |||
+ | Insect mouthparts and antennae are considered homologues of insect legs. Parallel developments are seen in some [[arachnids]]: The anterior pair of legs may be modified as analogues of antennae, particularly in [[whip scorpians]], which walk on six legs. These developments provide support for the theory that complex modifications often arise by duplication of components, with the duplicates modified in different directions. | ||
===Analogous structures and convergent evolution=== | ===Analogous structures and convergent evolution=== | ||
[[Image:Evolution eye.png|thumb|right|400px|'''Figure 6''': Inverted retina of vertebrate (left) and non-inverted retina of octopus (right)]] | [[Image:Evolution eye.png|thumb|right|400px|'''Figure 6''': Inverted retina of vertebrate (left) and non-inverted retina of octopus (right)]] | ||
− | Under similar environmental conditions, fundamentally different structures in different groups of organisms may undergo modifications to serve similar functions. This phenomenon is called [[convergent evolution]]. Similar structures, physiological processes or mode of life in organisms apparently bearing no close phylogenetic links but showing | + | Under similar environmental conditions, fundamentally different structures in different groups of organisms may undergo modifications to serve similar functions. This phenomenon is called [[convergent evolution]]. Similar structures, physiological processes or mode of life in organisms apparently bearing no close phylogenetic links but showing adaptations to perform the same functions are described as [[analogy|analogous]], for example: |
* Wings of [[bat]]s, [[bird]]s and [[insect]]s; | * Wings of [[bat]]s, [[bird]]s and [[insect]]s; | ||
* the jointed legs of [[insect]]s and [[vertebrate]]s; | * the jointed legs of [[insect]]s and [[vertebrate]]s; | ||
− | * | + | * tail [[fin]] of [[fish]], [[whale]] and [[lobster]]; |
* [[eye]]s of the [[vertebrate]]s and [[cephalopod]] molluscs ([[squid]] and [[octopus]]). Fig. 6 illustrates difference between an inverted and non-inverted [[retina]], the sensory cells lying beneath the [[nerve fibre]]s. This results in the sensory cells being absent where the [[optic nerve]] is attached to the eye, thus creating a [[blind spot]]. The octopus eye has a non-inverted retina in which the sensory cells lie above the nerve fibres. There is therefore no blind spot in this kind of eye. Apart from this difference the two eyes are remarkably similar, an example of convergent evolution. | * [[eye]]s of the [[vertebrate]]s and [[cephalopod]] molluscs ([[squid]] and [[octopus]]). Fig. 6 illustrates difference between an inverted and non-inverted [[retina]], the sensory cells lying beneath the [[nerve fibre]]s. This results in the sensory cells being absent where the [[optic nerve]] is attached to the eye, thus creating a [[blind spot]]. The octopus eye has a non-inverted retina in which the sensory cells lie above the nerve fibres. There is therefore no blind spot in this kind of eye. Apart from this difference the two eyes are remarkably similar, an example of convergent evolution. | ||
+ | |||
+ | {{seealso|Evolution of the eye}} | ||
===Vestigial organs=== | ===Vestigial organs=== | ||
− | + | ''Main article: [[Vestigial structure]]'' | |
− | A further aspect of comparative anatomy is the presence of vestigial organs. Organs that are smaller and simpler in structure than corresponding parts in the ancestral species are called vestigial organs. They are usually | + | A further aspect of comparative anatomy is the presence of vestigial organs. Organs that are smaller and simpler in structure than corresponding parts in the ancestral species are called vestigial organs. They are usually degenerated or underdeveloped. The existence of vestigial organs can be explained in terms of changes in the environment or modes of life of the species. Those organs are thought to be functional in the ancestral species but have now become unnecessary and non-functional. Examples are the vestigial hind limbs of whales, the [[haltere]] (vestigial hind [[wing]]s) of [[fly|flies]] and mosquitos, vestigial wings of flightless birds such as [[ostrich]]es, and the vestigial [[leaf|leaves]] of some [[xerophyte]]s (''e.g.'' [[cactus]]) and parasitic plants (''e.g.'' [[dodder]]). It must be noted however, that vestigial structures have lost the original function but may have another one. For example the halteres in [[dipterist]]s help balance the insect while in flight and the wings of ostriches are used in [[mating ritual]]s. |
==Evidence from geographical distribution== | ==Evidence from geographical distribution== | ||
Line 163: | Line 192: | ||
====Continental distribution==== | ====Continental distribution==== | ||
− | All organisms are adapted to their environment to a greater or lesser extent. If the abiotic and biotic factors within a [[habitat (ecology)|habitat]] are capable of supporting a particular species in one geographic area, then one might assume that the same species would be found in a similar habitat in a similar geographic area, e.g. in [[Africa]] and [[South America]]. This is not the case. Plant and animal species are discontinuously distributed throughout the | + | All organisms are adapted to their environment to a greater or lesser extent. If the abiotic and biotic factors within a [[habitat (ecology)|habitat]] are capable of supporting a particular species in one geographic area, then one might assume that the same species would be found in a similar habitat in a similar geographic area, e.g. in [[Africa]] and [[South America]]. This is not the case. Plant and animal species are discontinuously distributed throughout the world: |
* Africa has short-tailed ([[Old World]]) monkeys, [[elephant]]s, [[lion]]s and [[giraffe]]s. | * Africa has short-tailed ([[Old World]]) monkeys, [[elephant]]s, [[lion]]s and [[giraffe]]s. | ||
− | * South America has long-tailed monkeys, [[ | + | * South America has long-tailed monkeys, [[cougar]]s, [[jaguar]]s and [[llama]]s. |
− | + | Even greater differences can be found if [[Australia]] is taken into consideration though it occupies the same [[latitude]] as South America and Africa. [[Marsupial]]s like the [[kangaroo]] can be found in Australia, but are totally absent from Africa and are only represented by the [[opossum]] in [[South America]] and the [[Virginia Opossum]] in [[North America]]: | |
− | * | + | * The [[echidna]] and [[platypus]], the only living representatives of primitive egg-laying mammals ([[monotreme]]s), can be found only in Australia and are totally absent in the rest of the world. |
− | * On the other hand Australia has very few placental mammals except those that have been introduced by human. | + | * On the other hand, Australia has very few placental mammals except those that have been introduced by human beings. |
====Explanation==== | ====Explanation==== | ||
− | [[Image:Evolution con dis.png|thumb|right|450px|'''Figure | + | [[Image:Evolution con dis.png|thumb|right|450px|'''Figure 7''': Diagrams to the land bridge between continents in past geological time (A) and the barriers formed (B) due to the submergence of [[land bridge]]s.]] |
The main groups of modern mammal arose in [[Northern Hemisphere]] and subsequently migrated to three major directions: | The main groups of modern mammal arose in [[Northern Hemisphere]] and subsequently migrated to three major directions: | ||
− | * to South America via the [[land bridge]] in the [[Bering Strait]] and [[Isthmus of Panama]]; | + | * to South America via the [[land bridge]] in the [[Bering Strait]] and [[Isthmus of Panama]]; A large number of families of South American marsupials became extinct as a result of competition with these North American counterparts. |
− | *to Africa via the [[Strait of Gibraltar]]; and | + | * to Africa via the [[Strait of Gibraltar]]; and |
− | * | + | * to Australia via South East Asia to which it was at one time connected by land |
− | The shallowness of the Bering Strait would have made the passage of animals between two northern continents a | + | The shallowness of the Bering Strait would have made the passage of animals between two northern continents a relatively easy matter, and it explains the present-day similarity of the two [[fauna (animals)|fauna]]s. But once they had got down into the southern continents, they presumably became isolated from each other by various types of barriers. |
*'''The submerging of the Isthmus of Panama''': isolates the South American fauna | *'''The submerging of the Isthmus of Panama''': isolates the South American fauna | ||
*'''the [[Mediterranean Sea]] and the North African desert''': partially isolate the African fauna; and | *'''the [[Mediterranean Sea]] and the North African desert''': partially isolate the African fauna; and | ||
*'''the submerging of the original connection between Australia and South East Asia''': isolates the Australian fauna | *'''the submerging of the original connection between Australia and South East Asia''': isolates the Australian fauna | ||
− | Once isolated, the | + | Once isolated, the animals in each continent have shown adaptive radiation (Fig. 7) to evolve along their own lines. |
====Evidence for migration and isolation==== | ====Evidence for migration and isolation==== | ||
− | [[Image: | + | [[Image:Evolution evi mig.png|450px|thumb|right|Map of the world showing distribution of present members of camel. Solid black lines indicate possible migration routes.]] |
− | The fossil record for the [[camel]] indicated that evolution of camels started in | + | The fossil record for the [[camel]] indicated that evolution of camels started in North America, from which they migrated across the Bering Strait into Asia and hence to Africa, and through the Isthmus of Panama into South America. Once isolated, they evolved along their own lines, giving the modern camel in Asia and Africa and llama in South America. |
====Continental drift==== | ====Continental drift==== | ||
+ | The same kinds of fossils are found from areas known to be adjacent to one another in the past but which, through the process of [[continental drift]], are now in widely divergent geographic locations. For example, fossils of the same types of ancient amphibians, arthropods and ferns are found in South America, Africa, India, Australia and Antarctica, which can be dated to the [[Paleozoic Era]], at which time these regions were united as a single landmass called [[Gondwana]]. [http://biology.clc.uc.edu/courses/bio303/contdrift.htm] Sometimes the descendants of these organisms can be identified and show unmistakable similarity to each other, even though they now inhabit very different regions and climates. | ||
+ | |||
====Oceanic island distribution==== | ====Oceanic island distribution==== | ||
+ | Most small isolated islands only have native species that could have arrived by air or water; like birds, insects and turtles. The few large mammals present today were brought by human settlers in boats. Plant life on remote and recent volcanic islands like Hawaii could have arrived as airborne spores or as seeds in the droppings of birds. After the explosion of Krakatoa a century ago and the emergence of a steaming, lifeless remnant island called Anak Krakatoa (child of Krakatoa), plants arrived within months and within a year there were moths and spiders that had arrived by air. The island is now ecologially hard to distinguish from those around it that have been there for millions of years. | ||
− | ==Evidence from comparative | + | == Evidence from comparative physiology and biochemistry == |
− | ==Evidence from | + | :''See also:'' ''[[Archaeogenetics]], [[Common descent]], [[Last universal ancestor]], [[Most recent common ancestor]],'' [[Nothing in Biology Makes Sense Except in the Light of Evolution]], ''[[Speciation]], [[Timeline of evolution]], [[Timeline of human evolution]], [[Universal Code (Biology)]]'' |
− | === | + | |
− | === | + | === Evolution of widely distributed proteins and molecules === |
− | ==== | + | |
− | ==== | + | All known extant organisms make use of [[DNA]] and/or [[RNA]]. [[Adenosine triphosphate|ATP]] is used as metabolic currency by all extant life. The [[Genetic code]] is the same for almost every organism, meaning that a piece of [[RNA]] in a [[bacteria|bacterium]] codes for the same protein as in a human [[cell (biology)|cell]]. |
− | ==== | + | |
+ | A classic example of biochemical evidence for evolution is the variance of the [[protein]] [[Cytochrome c]] in living cells. The variance of cytochrome c of different organisms is measured in the number of differing [[amino acid]]s, each differing amino acid being a result of a base pair substitution, a [[mutation]]. If each differing amino acid is assumed to be the result of '''one''' base pair substitution, it can be calculated how long ago the two species diverged by multiplying the number of base pair substitutions by the estimated time it takes for a substituted base pair of the cytochrome c gene to be successfully passed on. For example, if the average time it takes for a base pair of the cytochrome c gene to mutate is N years, the number of amino acids making up the cytochrome c protein in monkeys differ by one from that of humans, this leads to the conclusion that the two species diverged N years ago. | ||
+ | |||
+ | Comparison of the DNA sequences allows organisms to be grouped by sequence similarity, and the resulting [[phylogenetics|phylogenetic]] trees are typically congruent with traditional taxonomy, and are often used to strengthen or correct taxonomic classifications. Sequence comparison is considered a measure robust enough to be used to correct erroneous assumptions in the phylogenetic tree in instances where other evidence is scarce. For example, neutral human DNA sequences are approximately 1.2% divergent (based on substitutions) from those of their nearest genetic relative, the [[chimpanzee]], 1.6% from [[gorilla]]s, and 6.6% from [[baboon]]s.<ref>Two sources: 'Genomic divergences between humans and other hominoids and the effective population size of the common ancestor of humans and chimpanzees'. and 'Quantitative Estimates of Sequence Divergence for Comparative Analyses of Mammalian Genomes' "[http://www.ncbi.nlm.nih.gov/entrez/query.fcgi?cmd=Retrieve&db=pubmed&dopt=Abstract&list_uids=11170892] [http://www.genome.org/cgi/content/full/13/5/813]"</ref> Genetic sequence evidence thus allows inference and quantification of genetic relatedness between humans and other apes.<ref>The picture labeled "Human Chromosome 2 and its analogs in the apes" in the article [http://www.gate.net/~rwms/hum_ape_chrom.html Comparison of the Human and Great Ape Chromosomes as Evidence for Common Ancestry] is literally a picture of a link in humans that links two separate chromosomes in the nonhuman apes creating a single chromosome in humans. It is considered a missing link, and the ape-human connection is of particular interest. Also, while the term originally referred to fossil evidence, this too is a trace from the past corresponding to some living beings which when alive were the physical embodiment of this link.</ref><ref>The [[New York Times]] report ''[http://www.nytimes.com/2006/03/07/science/07evolve.html Still Evolving, Human Genes Tell New Story]'', based on ''[http://biology.plosjournals.org/perlserv/?request=get-document&doi=10.1371/journal.pbio.0040072 A Map of Recent Positive Selection in the Human Genome]'', states the [[International HapMap Project]] is "providing the strongest evidence yet that humans are still evolving" and details some of that evidence.</ref> The sequence of the 16S [[rRNA]] gene, a vital gene encoding a part of the [[ribosome]], was used to find the broad phylogenetic relationships between all extant life. The analysis, originally done by [[Carl Woese]], resulted in the [[three-domain system]], arguing for two major splits in the early evolution of life. The first split led to modern [[Bacteria]] and the subsequent split led to modern [[Archaea]] and [[Eukaryote]]. | ||
+ | |||
+ | The [[proteome|proteomic]] evidence also supports the universal ancestry of life. Vital [[protein]]s, such as the [[ribosome]], [[DNA polymerase]], and [[RNA polymerase]], are found in everything from the most primitive bacteria to the most complex mammals. The core part of the protein is conserved across all lineages of life, serving similar functions. Higher organisms have evolved additional [[protein subunit]]s, largely affecting the regulation and [[protein-protein interaction]] of the core. Other overarching similarities between all lineages of extant organisms, such as [[DNA]], [[RNA]], [[amino acid]]s, and the [[lipid bilayer]], give support to the theory of common descent. The [[chirality (chemistry)|chirality]] of DNA, RNA, and amino acids is conserved across all known life. As there is no functional advantage to right- or left-handed molecular chirality, the simplest hypothesis is that the choice was made randomly by early organisms and passed on to all extant life through common descent. Further evidence for reconstructing ancestral lineages comes from [[junk DNA]] such as [[pseudogene]]s, "dead" genes which steadily accumulate mutations.<ref>Pseudogene evolution and natural selection for a compact genome. "[http://www.ncbi.nlm.nih.gov/entrez/query.fcgi?cmd=Retrieve&db=pubmed&dopt=Abstract&list_uids=10833048]"</ref> | ||
+ | |||
+ | There is also a large body of molecular evidence for a number of different mechanisms for large evolutionary changes, among them: genome and [[gene duplication]], which facilitates rapid evolution by providing substantial quantities of genetic material under weak or no selective constraints; [[horizontal gene transfer]], the process of transferring genetic material to another cell that is not an organism's offspring, allowing for species to acquire beneficial genes from each other; and [[genetic recombination|recombination]], capable of reassorting large numbers of different alleles and of establishing [[reproductive isolation]]. The [[Endosymbiotic theory]] explains the origin of [[mitochondrion|mitochondria]] and [[plastids]] (''e.g.'' [[chloroplast]]s), which are [[organelle]]s of eukaryotic cells, as the incorporation of an ancient [[prokaryote|prokaryotic]] cell into ancient [[eukaryote|eukaryotic]] cell. Rather than evolving [[eukaryote|eukaryotic]] [[organelle]]s slowly, this theory offers a mechanism for a sudden evolutionary leap by incorporating the genetic material and biochemical composition of a separate species. Evidence supporting this mechanism has recently been found in the [[protist]] [[Hatena]]: as a predator it engufes a green algae cell, which subsequently behaves as an [[endosymbiont]], nourishing Hatena, which in turn loses it's feeding apparatus and behaves as an [[autotroph]].<ref>{{cite journal| author = Okamoto N, Inouye I. | year =2005| title = A secondary symbiosis in progress| journal =Science | volume =310 | issue =5746 | pages =287}}</ref><ref>{{cite journal| author = Okamoto N, Inouye I. | year =2006| title = Hatena arenicola gen. et sp. nov., a Katablepharid Undergoing Probable Plastid Acquisition.| journal =Protist |volume = Article in Print}}</ref> | ||
+ | |||
+ | Since [[metabolism|metabolic]] processes do not leave fossils, research into the evolution of the basic cellular processes is done largely by comparison of existing organisms. Many lineages diverged when new metabolic processes appeared, and it is theoretically possible to determine when certain metabolic processes appeared by comparing the traits of the descendants of a common ancestor or by detecting their physical manifestations. As an example, the appearance of oxygen in the earth's atmosphere is linked to the evolution of photosynthesis. | ||
+ | |||
+ | ===Out of Africa hypothesis of human evolution=== | ||
+ | Mathematical models of evolution, pioneered by the likes of [[Sewall Wright]], [[Ronald Fisher]] and [[J. B. S. Haldane]] and extended via [[diffusion equation|diffusion theory]] by [[Motoo Kimura]], allow predictions about the genetic structure of evolving populations. Direct examination of the genetic structure of modern populations via DNA sequencing has recently allowed verification of many of these predictions. For example, the [[Recent single-origin hypothesis|Out of Africa]] theory of human origins, which states that modern humans developed in Africa and a small sub-population migrated out (undergoing a [[population bottleneck]]), implies that modern populations should show the signatures of this migration pattern. Specifically, post-bottleneck populations (Europeans and Asians) should show lower overall genetic diversity and a more uniform distribution of allele frequencies compared to the African population. Both of these predictions are borne out by actual data from a number of studies.{{Fact|date=February 2007}} | ||
+ | |||
+ | ===External link=== | ||
+ | * [http://www.talkorigins.org/faqs/comdesc/section4.html Evidences for Macroevolution, Part 4: The Molecular Sequence Evidence] | ||
+ | |||
+ | ==Evidence from antibiotic and pesticide resistance== | ||
+ | The development and spread of [[antibiotic resistance|antibiotic resistant]] [[bacteria]], like the spread of [[pesticide]] resistant forms of plants and insects is evidence for evolution of species, and of change within species. Thus the appearance of [[vancomycin]] resistant [[Golden staph|Staphlococcus aureus]], and the danger it poses to hospital patients is a direct result of evolution through natural selection. Similarly the appearance of DDT resistance in various forms of [[Anopheles]] mosqitoes, and the appearance of [[myxomatosis]] resistance in breeding rabbit populations in Australia, are all evidence of the existence of evolution in situations of evolutionary [[selection pressure]] in species in which generations occur rapidly. | ||
+ | |||
+ | ==Evidence from studies of complex iteration== | ||
+ | "It has taken more than five decades, but the electronic computer is now powerful enough to simulate evolution" [http://www.trnmag.com/Stories/2003/052103/Simulated_evolution_gets_complex_052103.html] assisting [[bioinformatics]] in its attempt to solve biological problems. | ||
+ | |||
+ | [[Computer science]] allows the [[iteration]] of self changing [[complex system]]s to be studied, allowing a mathematically exact understanding of the nature of the processes behind evolution; providing evidence for the hidden causes of known evolutionary events. The evolution of specific cellular mechanisms like [[spliceosome]]s that can turn the cell's genome into a vast workshop of billions of interchangeable parts that can create tools that create tools that create tools that create us can be studied for the first time in an exact way. | ||
+ | |||
+ | For example, Christoph Adami ''et al.'' make this point in ''Evolution of biological complexity'': | ||
+ | :To make a case for or against a trend in the evolution of complexity in biological evolution, complexity needs to be both rigorously defined and measurable. A recent information-theoretic (but intuitively evident) definition identifies genomic complexity with the amount of information a sequence stores about its environment. We investigate the evolution of genomic complexity in populations of digital organisms and monitor in detail the evolutionary transitions that increase complexity. We show that, because natural selection forces genomes to behave as a natural "[[Maxwell's Demon|Maxwell Demon]]," within a fixed environment, genomic complexity is forced to increase. [http://www.pnas.org/cgi/content/full/97/9/4463] | ||
+ | |||
+ | For example, David J. Earl and Michael W. Deem make this point in ''Evolvability is a selectable trait'': | ||
+ | :Not only has life evolved, but life has evolved to evolve. That is, correlations within protein structure have evolved, and mechanisms to manipulate these correlations have evolved in tandem. The rates at which the various events within the hierarchy of evolutionary moves occur are not random or arbitrary but are selected by Darwinian evolution. Sensibly, rapid or extreme environmental change leads to selection for greater evolvability. This selection is not forbidden by causality and is strongest on the largest-scale moves within the mutational hierarchy. Many observations within evolutionary biology, heretofore considered evolutionary happenstance or accidents, are explained by selection for evolvability. For example, the vertebrate immune system shows that the variable environment of antigens has provided selective pressure for the use of adaptable codons and low-fidelity polymerases during [[somatic hypermutation]]. A similar driving force for biased codon usage as a result of productively high mutation rates is observed in the hemagglutinin protein of [[Influenzavirus A|influenza A]]. [http://www.pnas.org/cgi/content/full/101/32/11531] | ||
+ | |||
+ | "Computer simulations of the evolution of linear sequences have demonstrated the importance of recombination of blocks of sequence rather than point mutagenesis alone. Repeated cycles of point mutagenesis, recombination, and selection should allow ''in vitro'' molecular evolution of complex sequences, such as proteins." [http://www.pubmedcentral.nih.gov/articlerender.fcgi?artid=45099] Evolutionary molecular engineering, also called directed evolution or ''in vitro'' molecular evolution involves the iterated cycle of mutation, multiplication with recombination, and selection of the fittest of individual molecules (proteins, DNA, and RNA). Natural evolution can be relived showing us possible paths from catalytic cycles based on proteins to based on RNA to based on DNA. [http://www.scripps.edu/newsandviews/e_20060327/evo.html] | ||
+ | [http://bio.kaist.ac.kr/~jsrhee/research03.html] [http://www.isgec.org/gecco-2005/free-tutorials.html#ivme] [http://www.pubmedcentral.nih.gov/articlerender.fcgi?artid=45099] | ||
+ | |||
+ | ==Evidence from speciation== | ||
+ | ===Hawthorn fly=== | ||
+ | |||
+ | One example of evolution at work is the case of the hawthorn fly, ''[[Rhagoletis pomonella]]'', also known as the apple maggot fly, which appears to be undergoing [[sympatric speciation]].<ref>{{cite journal| author =Feder ''et al'' | year =2003| title = Evidence for inversion polymorphism related to sympatric host race formation in the apple maggot fly, Rhagoletis pomonella.| journal =Genetics | volume =163 | issue =3|pages =939-953}}</ref> Different populations of hawthorn fly feed on different fruits. A distinct population emerged in North America in the 19th century some time after [[apples]], a non-native species, were introduced. This apple-feeding population normally feeds only on apples and not on the historically preferred fruit of [[Crataegus|hawthorns]]. The current hawthorn feeding population does not normally feed on apples. Some evidence, such as the fact that six out of thirteen [[allozyme]] loci are different, that hawthorn flies mature later in the season and take longer to mature than apple flies; and that there is little evidence of interbreeding (researchers have documented a 4-6% hybridization rate) suggests that this is occurring. The emergence of the new hawthorn fly is an example of evolution in progress.<ref>Berlocher, S.H. and G.L. Bush. 1982. An electrophoretic analysis of Rhagoletis (Diptera: Tephritidae) phylogeny. Systematic Zoology 31:136-155; Berlocher, S.H. and J.L. Feder. 2002. Sympatric speciation in phytophagous insects: moving beyond controversy? Annual Review of Entomology 47:773-815; Bush, G.L. 1969. Sympatric host race formation and speciation in frugivorous flies of the genus Rhagoletis (Diptera: Tephritidae). Evolution 23:237-251; Prokopy, R.J., S.R. Diehl and S.S. Cooley. 1988. Behavioral evidence for host races in Rhagoletis pomonella flies. [[Oecologia]] 76:138-147. [http://www.pnas.org/cgi/content/full/94/21/11417 Proc. Natl. Acad. Sci. USA - Vol. 94, pp. 11417-11421, October 1997 - Evolution] article Selective maintenance of allozyme differences among sympatric host races of the apple maggot fly.</ref> | ||
==See also== | ==See also== | ||
+ | *[[Human history]] | ||
+ | *[[Objections to evolution]] | ||
==References== | ==References== | ||
− | *Darwin, Charles [[November 24]] [[1859]]. ''On the [[Origin of Species]] by means of Natural Selection or the Preservation of Favoured Races in the Struggle for Life''. London: John Murray, Albemarle Street. 502 pages. Reprinted: Gramercy (May 22, 1995). ISBN | + | <references/> |
− | *Mayr, Ernst. ''What Evolution Is''. Basic Books (October, 2002). ISBN | + | *Darwin, Charles [[November 24]] [[1859]]. ''On the [[Origin of Species]] by means of Natural Selection or the Preservation of Favoured Races in the Struggle for Life''. London: [[John Murray (publisher)|John Murray]], [[Albemarle Street]]. 502 pages. Reprinted: Gramercy (May 22, 1995). ISBN 0-517-12320-7 |
− | *Gigerenzer, Gerd, et al., ''The empire of chance: how probability changed science and everyday life'' (New York: Cambridge University Press, 1989). | + | *Mayr, Ernst. ''What Evolution Is''. Basic Books (October, 2002). ISBN 0-465-04426-3 |
+ | *Gigerenzer, Gerd, ''et al.'', ''The empire of chance: how probability changed science and everyday life'' (New York: Cambridge University Press, 1989). | ||
*Williams, G.C. (1966). Adaptation and Natural Selection: A Critique of some Current Evolutionary Thought. Princeton, N.J.: Princeton University Press. | *Williams, G.C. (1966). Adaptation and Natural Selection: A Critique of some Current Evolutionary Thought. Princeton, N.J.: Princeton University Press. | ||
*''Biological science'', Oxford, 2002. | *''Biological science'', Oxford, 2002. | ||
*CJ Clegg, 1999, ''Genetics and Evolution'', John Murray. ISBN 0-7195-7552-4 | *CJ Clegg, 1999, ''Genetics and Evolution'', John Murray. ISBN 0-7195-7552-4 | ||
*Y.K. Ho, 2004, ''Advanced-level Biology for Hong Kong'', Manhattan Press. ISBN 962-990-635-X | *Y.K. Ho, 2004, ''Advanced-level Biology for Hong Kong'', Manhattan Press. ISBN 962-990-635-X | ||
+ | *Paul, Christopher R. C. (1998) ''The Adequacy of the Fossil Record'', John Wiley & Sons, ISBN 0-471-96988-5 | ||
+ | *Behrensmeyer, Anna K. (1980) ''Fossils in the making: Vertebrate taphonomy and paleoecology'', University of Chicago Press, ISBN 0-226-04169-7 | ||
+ | *Martin, Ronald E. ''et al.'' eds. (1999) ''Taphonomy: A Process Approach'', Cambridge University Press, ISBN 0-521-59833-8 | ||
==External links== | ==External links== | ||
+ | * [http://www.talkorigins.org/faqs/comdesc/ 29+ Evidences for Macroevolution: The Scientific Case for Common Descent] | ||
+ | * [http://www.trueorigin.org/theobald1a.asp A critique of the aforementioned 29+ Evidences for Macroevolution] | ||
+ | * [http://www.talkorigins.org/faqs/comdesc/camp.html Response to the "critique"] | ||
* [http://nationalacademies.org/evolution/ National Academies Evolution Resources] | * [http://nationalacademies.org/evolution/ National Academies Evolution Resources] | ||
* [http://www.chains-of-reason.org/chains/evolution-by-natural-selection/introduction.htm Evolution by Natural Selection] — An introduction to the logic of the theory of evolution by natural selection | * [http://www.chains-of-reason.org/chains/evolution-by-natural-selection/introduction.htm Evolution by Natural Selection] — An introduction to the logic of the theory of evolution by natural selection | ||
Line 219: | Line 297: | ||
* [http://www.genomenewsnetwork.org/categories/index/genome/evolution.php Evolution News from Genome News Network (GNN)] | * [http://www.genomenewsnetwork.org/categories/index/genome/evolution.php Evolution News from Genome News Network (GNN)] | ||
* [http://www.nap.edu/books/0309063647/html/ National Academy Press: Teaching About Evolution and the Nature of Science] | * [http://www.nap.edu/books/0309063647/html/ National Academy Press: Teaching About Evolution and the Nature of Science] | ||
+ | * [http://www.talkorigins.org/faqs/faq-transitional.html Transitional Vertebrate Fossils FAQ] | ||
+ | |||
+ | {{evolution}} | ||
− | {{credit| | + | {{credit|Evidence_of_common_descent|138267681}} |
[[Category:Life sciences]] | [[Category:Life sciences]] |
Revision as of 17:57, 16 June 2007
Outline:
Evidences of evolution refers generally to those evidences of evolution by common descent and sometimes also evolution by natural selection.
Darwin's theory of evolution encompasses several distinction theories, but primarily two main ones: the theory of descent with modificaiton and the theory of natural selection. The theory of descent.... The theory of natural ....
In a broad sense, evolutoin referes to changes in gene frequencies over ... generations.... Thus, any observed change in gene frequencies... , such as the development of antibiotic resistence in bacterial, constittues evidens of evolution. More generally, however, evolution is used i the sense of TDM... sometimes TDM and TNS.
This article will deal with evidences for these two main theories on the macroevotuionary level. Marcorevolutoin..... Microevolution....
refers to changes in gene frequencies .....
Notes: Broadly defined, evolution is any heritable change in a population of organisms over time. Changes may be slight or large, but must be passed on to the next generation (or many generations) and must involve populations, not individuals. A popular definition along these lines is that offered by Douglas J. Futuyama (1986) in Evolutionary Biology: "Biological evolution…is change in the properties of populations of organisms that transcend the lifetime of a single individual…. The changes in populations that are considered evolutionary are those that are inheritable via the genetic material from one generation to another."
Similarly, the term may be presented in terms of allele frequency (with an "allele" being an alternative form of a gene, such as different alleles code for different eye colors): "Evolution can be precisely defined as any change in the frequency of alleles within a gene pool from one generation to the next" (Curtis & Barnes, 1989). Both a slight change (as in pesticide resistance in a strain of bacteria) and a large change (as in the development of major new designs such as feathered wings for flying, or even the present diversity of life from simple prokaryotes) qualify as evolution.
However, the term evolution is often used with more narrow meanings. It is not uncommon to see the term equated to the specific theory that all organisms have descended from common ancestors (which is also known as the "theory of descent with modification"). Less frequently, evolution is sometimes used to refer to one explanation for the process by which change occurs, the "theory of modification through natural selection." In addition, the term evolution is occasionally used with reference to a comprehensive theory that includes both the non-causal pattern of descent with modification and the causal mechanism of natural selection.
As broadly and commonly defined in the scientific community, the term evolution connotes heritable changes in populations of organisms over time, or changes in the frequencies of alleles over time. In this sense, the term does not specify any overall pattern of change through the ages, nor the process whereby change occurs (although the term is also employed in such a manner).
However, there are two very important and popular evolutionary theories that address the pattern and process of evolution: "theory of descent with modification" and "theory of natural selection," respectively, as well as other concepts in evolutionary theory that deal with speciation and the rate of evolution.
Theory of descent with modification
The "theory of descent with modification" is the major kinematic theory that deals with the pattern of evolution—that is, it treats non-causal relations between ancestral and descendent species, orders, phyla, and so forth. The theory of descent with modification, also called the "theory of common descent," essentially postulates that all organisms have descended from common ancestors by a continuous process of branching. In other words, all life evolved from one kind of organism or from a few simple kinds, and each species arose in a single geographic location from another species that preceded it in time. Each group of organisms shares a common ancestor.
One of the major contributions of Charles Darwin was to marshal substantial evidence for the theory of descent with modification, particularly in his book, Origin of Species. Among the evidences that evolutionists use to document the "pattern of evolution" are the fossil record, the distribution patterns of existing species, methods of dating fossils, and comparison of homologous structures. (See Evidences of Evolution below.)
Theory of natural selection
Main articles: Darwinism and Natural selection
The second major evolutionary theory is the "theory of modification through natural selection," also known as the "theory of natural selection." This is a dynamic theory that involves mechanisms and causal relationships. The theory of natural selection is one explanation offered for how evolution might have occurred; in other words, the "process" by which evolution took place to arrive at the pattern.
Natural selection may be defined as the mechanism whereby biological individuals that are endowed with favorable or deleterious traits reproduce more or less than other individuals that do not possess such traits.
According to this theory, natural selection is the directing or creative force of evolution.
Note: This is only a very rough draft, with notes that may be useful in developing the article. Please do not edit this article until the actual article is complete — i.e., when this notice is removed. You may add comments on what you would like to see included. Rick Swarts 00:05, 28 Sep 2005 (UTC)
In Darwin's comprehensive theory of evolution, there can actually be elucidated at least five major, largely independent theories. The two basic theories, and the ones which I will treat here, are: (1) the theory of evolution by common descent, and (2) the theory of modification through natural selection. The first is a kinematic theory which deals with non-causal relations between things — it deals with the pattern of evolution. The latter is a dynamic theory which deals with mechanisms and causal relationships — it deals with the process. Other theories offered by Darwin deal with (3) evolution as such (the fact of evolution), (4) the gradualness of evolution, and (5) populational speciation.
The "theory of descent with modification" essentially postulates that all organisms have descended from common ancestors by a continuous process of branching. In other words, all life evolved from one kind of organism or from a few simple kinds, and each species arose in a single geographic location, from another species that preceded it in time. Evolutionists have marshaled substantial evidence for the theory of descent with modification. That is, the "pattern of evolution" is well documented by the fossil record, the distribution patterns of existing species, methods of dating fossils, and comparison of homologous structures. Interestingly, all of the classical arguments for evolution are fundamentally arguments for imperfections that reflect history. They fit the pattern of observing that the leg of Reptile B is not the best for walking, because it evolved from Fish A. In other words, why would a rat run, a bat fly, a porpoise swim and a man type all with the same structures utilizing the same bones unless inherited from a common ancestor?
Evidence is so overwhelming for the theory of descent with modification that only religious fundamentalists have attempted to challenge this theory. Among these are the “scientific creationists.” Scientific creationists are a specific group of creationists who maintain that modern organisms did not descend from common ancestors, and that their only historical connectedness is in the mind of God. Instead, scientific creationists promulgate the view that living organisms are immutable, and were all created by God in a short time period, on a earth whose age is generally measured in 1000s of years. The substantial fossil record is dismissed in various ways, including as a trick of God and as an artifact from the Great Flood (with some organisms sinking faster than others and thus on a lower fossil plane). Although some individual presentations by scientific creationists are quite sophisticated, the overall theory of scientific creationism runs counter to an enormous body of evidence and thus is strongly criticized by most of the scientific community.
The second theory of Darwin, the "theory of modification through natural selection," is one explanation offered for how evolution might have occurred, i.e, the "process" by which evolution took place and arrived at the pattern. This theory of natural selection was the most revolutionary and controversial concept advanced by Darwin. While the theory of descent with modification was accepted soon after its introduction, the theory of natural selection took until the mid-1900s to be accepted by the scientific community. By providing a purely non-teleogical, materialistic explanation for all phenomenon of living nature, it was said it "dethroned God." According to this theory, natural selection is the directing or creative force of evolution. Natural selection is considered far more than just a minor force for weeding out unfit organisms. Even Paley and other natural theologians accepted natural selection, albeit as a devise for removing unfit organisms, rather than as a directive force for creating new species and new designs. It is this theory of natural selection that has the three radical components mentioned earlier: (a) purposelessness (no higher purpose, just the struggle of individuals to survive and reproduce); (b) philosophical materialism (matter is seen as the ground of all existence with spirit and mind being produced by or a function of the material brain); and (c) the view that evolution is not progressive from lower to higher, but just an adaptation to local environments; it could form a man with his superior brain or a parasite, but no one could say which is higher or lower.
Concrete evidence for the theory of modification by natural selection is limited to microevolution, such as seen in the case of artificial selection, whereby various breeds of animals and varieties of plants have been produced that are different in some respect from their ancestors, or in the often-cited, but somewhat problematic case of systematic color change in the peppered moth, Biston betularia, which was observed over a 50-year period in England. The evidence that natural selection directs the major transitions between species and originates new designs (macroevolution) necessarily involves extrapolation from these evidences on the microevolutionary level. That is, it is inferred that if moths can change their color in 50 years, then new designs or entire new genera can originate over millions of years. If geneticists see population changes for fruit flies in laboratory bottles, then given eons of time, birds can be built from reptiles and fish with jaws from jawless ancestors. One of Darwin's chief purposes in publishing the Origin of Species was to show that natural selection had been the chief agent of the change presented in the theory of descent with modification. The validity of making this extrapolation has recently come under strong challenge from top evolutionists.
The issue has been further complicated by the fact that textbooks have persisted in presenting some proofs for evolution which are false or misleading, as pointed out by Jonathan Wells in his book Icons of Evolution. These widely-known but misleading teachings include the famous Miller –Urey experiment in which sparks are sent through a mixture of gases and yield the building blocks of amino acids, and the drawings by Ernst Haeckel of the early embryonic stages of such vertebrates as fish, chick, rabbits and humans, whereby it is exhibited that the earliest stages in all of these vertebrates are virtually identical. Wells reports that scientists have known for years both that the Miller-Urey experiments did not really approximate conditions of the early earth and that Haeckel had faked his drawings, since in reality the vertebrate embryos never look as similar as he made them look. These errors are well-known, yet textbook authors persist in using these examples. Another interesting case is the classic example of natural selection as seen in the case of the peppered moth (Biston belularia) in England, known as a case of industrial melanism, whereby a shift toward darker melanic forms is seen and attributed to an heightened predation by birds of the light-colored moths, because the lighter forms could more easily be seen on the tree trunks which have been increasingly darkened from pollution. In these cases, individuals have known that peppered moths do not normally alight on tree trunks, and there are even inverse correlations with pollution in many situations. Textbook photos are generally staged by gluing or pinning the moths to tree trunks. Some authors have responded that they knew the peppered moth case had problems, but they were good examples because they were easily grasped by the students. Use of such flawed cases has the unfortunate consequence of causing distrust of science by the students.
Evidence is so overwhelming for the theory of descent with modification that only religious fundamentalists have attempted to challenge this theory. Among these are the Ascientific creationists.@ Scientific creationists@ are a specific group of creationists who maintain that modern organisms did not descend from common ancestors, and that their only historical connectedness is in the mind of God. Instead, scientific creationists promulgate the view that living organisms are immutable, and were all created by God in a short time period, on a earth whose age is generally measured in 1000s of years. The substantial fossil record is dismissed in various ways, including as a trick of God and as an artifact from the Great Flood (with some organisms sinking faster than others and thus on a lower fossil plane). Although some individual presentations by scientific creationists are quite sophisticated, the overall theory of scientific creationism runs counter to an enormous body of evidence and thus is strongly criticized by most of the scientific community.
In reality, most evidences that are presented are actually for the theory of descent with modification. Concrete evidence for the theory of modification by natural selection is limited to microevolution — that is, within populations or species. For example, it is observed in the increased pesticide resistance in a species of bacteria. Artificial selection within populations or species also provides evidence, such as in the production of various breeds of animals by selective breeding, or and varieties of plants by selective cultivation. However, the evidence that natural selection directs changes on the macroevolution the major transitions between species and originates new designs (macroevolution) necessarily involves extrapolation from these evidences on the microevolutionary level. That is, it is inferred that if moths can change their color in 50 years, then new designs or entire new genera can originate over millions of years. If geneticists see population changes for fruit flies in laboratory bottles, then given eons of time, birds can be built from reptiles and fish with jaws from jawless ancestors. One of Darwin's chief purposes in publishing the Origin of Species was to show that natural selection had been the chief agent of the change presented in the theory of descent with modification. The validity of making this extrapolation has recently come under strong challenge from top evolutionists.
The wide range of evidence of evolution provides a wealth of information on the natural processes by which the variety of life on Earth developed.
Fossils are important for estimating when various lineages developed. As fossilization is an uncommon occurrence, usually requiring hard body parts and death near a site where sediments are being deposited, the fossil record only provides sparse and intermittent information about the evolution of life. Evidence of organisms prior to the development of hard body parts such as shells, bones and teeth is especially scarce, but exists in the form of ancient microfossils, as well as impressions of various soft-bodied organisms.
Comparison of the genetic sequence of organisms has revealed that organisms that are phylogenetically close have a higher degree of sequence similarity than organisms that are phylogenetically distant. Further evidence for common descent comes from genetic detritus such as pseudogenes, regions of DNA that are orthologous to a gene in a related organism, but are no longer active and appear to be undergoing a steady process of degeneration. Since metabolic processes do not leave fossils, research into the evolution of the basic cellular processes is also done largely by comparison of existing organisms. Many lineages diverged at different stages of development, so it is theoretically possible to determine when certain metabolic processes appeared by comparing the traits of the descendants of a common ancestor.
Evidence from paleontology
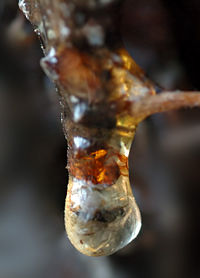
When organisms die, they often decompose rapidly or are consumed by scavengers, leaving no permanent evidences of their existence. However, occasionally, some organisms are preserved. The remains or traces of organisms from a past geologic age embedded in rocks by natural processes are called fossils. They are extremely important for understanding the evolutionary history of life on Earth, as they provide direct evidence of evolution and detailed information on the ancestry of organisms. Paleontology is the study of past life based on fossil records and their relations to different geologic time periods.
For fossilization to take place, the traces and remains of organisms must be quickly buried so that weathering and decomposition do not occur. Skeletal structures or other hard parts of the organisms are the most commonly occurring form of fossilized remains (Paul, 1998), (Behrensmeyer, 1980) and (Martin, 1999). There are also some trace "fossils" showing moulds, cast or imprints of some previous organisms.
As an animal dies, the organic materials gradually decay, such that the bones become porous. If the animal is subsequently buried in mud, mineral salts will infiltrate into the bones and gradually fill up the pores. The bones will harden into stones and be preserved as fossils. This process is known as petrification. If dead animals are covered by wind-blown sand, and if the sand is subsequently turned into mud by heavy rain or floods, the same process of mineral infiltration may occur. Apart from petrification, the dead bodies of organisms may be well preserved in ice, in hardened resin of coniferous trees (amber), in tar, or in anaerobic, acidic peat. Fossilization can sometimes be a trace, an impression of a form. Examples include leaves and footprints, the fossils of which are made in layers that then harden.
Fossil records
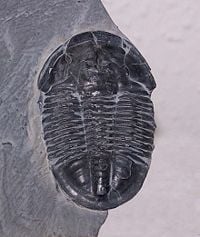
It is possible to find out how a particular group of organisms evolved by arranging its fossil records in a chronological sequence. Such a sequence can be determined because fossils are mainly found in sedimentary rock. Sedimentary rock is formed by layers of silt or mud on top of each other; thus, the resulting rock contains a series of horizontal layers, or strata. Each layer contains fossils which are typical for a specific time period during which they were made. The lowest strata contain the oldest rock and the earliest fossils, while the highest strata contain the youngest rock and more recent fossils.
A succession of animals and plants can also be seen from fossil records. Fossil evidence supports the theory that organisms tend to progressively increase in complexity. By studying the number and complexity of different fossils at different stratigraphic levels, it has been shown that older fossil-bearing rocks contain fewer types of fossilized organisms, and they all have a simpler structure, whereas younger rocks contain a greater variety of fossils, often with increasingly complex structures.
In the past, geologists could only roughly estimate the ages of various strata and the fossils found. They did so, for instance, by estimating the time for the formation of sedimentary rock layer by layer. Today, by measuring the proportions of radioactive and stable elements in a given rock, the ages of fossils can be more precisely dated by scientists. This technique is known as radiometric dating.
Throughout the fossil record, many species that appear at an early stratigraphic level disappear at a later level. This is interpreted in evolutionary terms as indicating the times at which species originated and became extinct. Geographical regions and climatic conditions have varied throughout the Earth's history. Since organisms are adapted to particular environments, the constantly changing conditions favoured species which adapted to new environments through the mechanism of natural selection.
According to fossil records, some modern species of plants and animals are found to be almost identical to the species that lived in ancient geological ages. They are existing species of ancient lineages that have remained morphologically (and probably also physiologically) somewhat unchanged for a very long time. Consequently, they are called "living fossils" by laypeople. Examples of "living fossils" include the tuatara, the nautilus, the horseshoe crab, the coelacanth, the ginkgo, the Wollemi pine, and the metasequoia.
Extent of the Fossil Record
Despite the relative rarity of suitable conditions for fossilization, approximately 250,000 fossil species are known[4]. The number of individual fossils this represents varies greatly from species to species, but many millions of fossils have been recovered: for instance, more than three million fossils from the last Ice Age have been recovered from the La Brea Tar Pits in Los Angeles[5]. Many more fossils are still in the ground, in various geological formations known to contain a high fossil density, allowing estimates of the total fossil content of the formation to be made. An example of this occurs in South Africa's Beaufort Formation (part of the Karoo Supergroup, which covers most of South Africa), which is rich in vertebrate fossils, including therapsids (reptile/mammal transitional forms)[6]. It has been estimated[7] that this formation contains 800 billion vertebrate fossils.
Evolution of the horse
- Further information: Evolution of the horse
Due to an almost-complete fossil record found in North American sedimentary deposits from the early Eocene to the present, the horse provides one of the best examples of evolutionary history (phylogeny).
This evolutionary sequence starts with a small animal called the Hyracotherium which lived in North America about 54 million years ago, then spread across to Europe and Asia. Fossil remains of Hyracotherium show it to have differed from the modern horse in three important respects: it was a small animal (the size of a fox), lightly built and adapted for running; the limbs were short and slender, and the feet elongated so that the digits were almost vertical, with four digits in the forelimbs and three digits in the hindlimbs; and the incisors were small, the molars having low crowns with rounded cusps covered in enamel.
The probable course of development of horses from Hyracotherium to Equus (the modern horse) involved at least 12 genera and several hundred species. The major trends seen in the development of the horse to changing environmental conditions may be summarized as follows:
- Increase in size (from 0.4 m to 1.5 m);
- Lengthening of limbs and feet;
- Reduction of lateral digits;
- Increase in length and thickness of the third digit;
- Increase in width of incisors;
- Replacement of premolars by molars; and
- Increases in tooth length, crown height of molars.
Fossilized plants found in different strata show that the marshy, wooded country in which Hyracotherium lived became gradually drier. Survival now depended on the head being in an elevated position for gaining a good view of the surrounding countryside, and on a high turn of speed for escape from predators, hence the increase in size and the replacement of the splayed-out foot by the hoofed foot. The drier, harder ground would make the original splayed-out foot unnecessary for support. The changes in the teeth can be explained by assuming that the diet changed from soft vegetation to grass. A dominant genus from each geological period has been selected to show the progressive development of the horse. However, it is important to note that there is no evidence that the forms illustrated are direct descendants of each other, even though they are related.
Limitations
The fossil record is an important source for scientists when tracing the evolutionary history of organisms. However, because of limitations inherent in the record, there are not fine scales of intermediate forms between related groups of species. This lack of continuous fossils in the record is a major limitation in tracing the descent of biological groups. Furthermore, there are also much larger gaps between major evolutionary lineages. These gaps are often referred to as "missing links".
There is a gap of about 100 million years between the early Cambrian period and the later Ordovician period. The early Cambrian period was the period from which numerous fossils of sponges, cnidarians (e.g., jellyfish), echinoderms (e.g., eocrinoids), molluscs (e.g., snails) and arthropods (e.g., trilobites) are found. In the later Ordovician period, the first animal that really possessed the typical features of vertebrates, the Australian fish, Arandaspis appeared. Thus few, if any, fossils of an intermediate type between invertebrates and vertebrates have been found, although likely candidates include the Burgess Shale animal, Pikaia gracilens, and its Maotianshan Shales relatives, Myllokunmingia, Yunnanozoon, Haikouella lanceolata, and Haikouichthys.
Some of the reasons for the incompleteness of fossil records are:
- In general, the probability that an organism becomes fossilized after death is very low;
- Some species or groups are less likely to become fossils because they are soft-bodied;
- Some species or groups are less likely to become fossils because they live (and die) in conditions that are not favourable for fossilization to occur in;
- Many fossils have been destroyed through erosion and tectonic movements;
- Some fossil remains are complete, but most are fragmentary;
- Some evolutionary change occurs in populations at the limits of a species' ecological range, and as these populations are likely to be small, the probability of fossilization is lower (see punctuated equilibrium);
- Similarly, when environmental conditions change, the population of a species is likely to be greatly reduced, such that any evolutionary change induced by these new conditions is less likely to be fossilized;
- Most fossils convey information about external form, but little about how the organism functioned;
- Using present-day biodiversity as a guide, this suggests that the fossils unearthed represent only a small fraction of the large number of species of organisms that lived in the past.
Evidence from comparative anatomy
Comparative study of the anatomy of groups of animals or plants reveals that certain structural features are basically similar. For example, the basic structure of all flowers consists of sepals, petals, stigma, style and ovary; yet the size, colour, number of parts and specific structure are different for each individual species.
Homologous structures and divergent (adaptive) evolution
If widely separated groups of organisms are originated from a common ancestry, they are expected to have certain basic features in common. The degree of resemblance between two organisms should indicate how closely related they are in evolution:
- Groups with little in common are assumed to have diverged from a common ancestor much earlier in geological history than groups which have a lot in common;
- In deciding how closely related two animals are, a comparative anatomist looks for structures that are fundamentally similar, even though they may serve different functions in the adult. Such structures are described as homologous and suggest a common origin.
- In cases where the similar structures serve different functions in adults, it may be necessary to trace their origin and embryonic development. A similar developmental origin suggests they are the same structure, and thus likely to be derived from a common ancestor.
When a group of organisms share a homologous structure which is specialized to perform a variety of functions in order to adapt different environmental conditions and modes of life are called adaptive radiation. The gradual spreading of organisms with adaptive radiation is known as divergent evolution.
Pentadactyl limb

The pattern of limb bones called pentadactyl limb is an example of homologous structures (Fig. 5a). It is found in all classes of tetrapods (i.e. from amphibians to mammals). It can even be traced back to the fins of certain fossil fishes from which the first amphibians are thought to have evolved. The limb has a single proximal bone (humerus), two distal bones (radius and ulna), a series of carpals (wrist bones), followed by five series of metacarpals (palm bones) and phalanges (digits). Throughout the tetrapods, the fundamental structures of pentadactyl limbs are the same, indicating that they originated from a common ancestor. But in the course of evolution, these fundamental structures have been modified. They have become superficially different and unrelated structures to serve different functions in adaptation to different environments and modes of life. This phenomenon is clearly shown in the forelimbs of mammals. For example:
- In the monkey, the forelimbs are much elongated to form a grasping hand for climbing and swinging among trees.
- In the pig, the first digit is lost, and the second and fifth digits are reduced. The remaining two digits are longer and stouter than the rest and bear a hoof for supporting the body.
- In the horse, the forelimbs are adapted for support and running by great elongation of the third digit bearing a hoof.
- The mole has a pair of short, spade-like forelimbs for burrowing.
- The anteater uses its enlarged third digit for tearing down ant hills and termite nests.
- In the whale, the forelimbs become flippers for steering and maintaining equilibrium during swimming.
- In the bat, the forelimbs have turned into wings for flying by great elongation of four digits, and the hook-like first digit remains free for hanging from trees.
Insect mouthparts
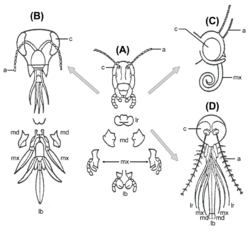
The basic structures are the same which include a labrum (upper lip), a pair of mandibles, a hypopharynx (floor of mouth), a pair of maxillae and a labium. These structures are enlarged and modified; others are reduced and lost. The modifications enable the insects to exploit a variety of food materials (Fig. 5b):
(A) Primitive state — biting and chewing: e.g. grasshopper. Strong mandibles and maxillae for manipulating food.
(B) Ticking and biting: e.g. honey bee. Labium long to lap up nectar; mandibles chew pollen and mould wax.
(C) Sucking: e.g. butterfly. Labrum reduced; mandibles lost; maxillae long forming sucking tube.
(D) Piercing and sucking, e.g. female mosquito. Labrum and maxillae form tube; mandibles form piercing stylets; labrum grooved to hold other parts.
Other Arthropod Appendages
Insect mouthparts and antennae are considered homologues of insect legs. Parallel developments are seen in some arachnids: The anterior pair of legs may be modified as analogues of antennae, particularly in whip scorpians, which walk on six legs. These developments provide support for the theory that complex modifications often arise by duplication of components, with the duplicates modified in different directions.
Analogous structures and convergent evolution
Under similar environmental conditions, fundamentally different structures in different groups of organisms may undergo modifications to serve similar functions. This phenomenon is called convergent evolution. Similar structures, physiological processes or mode of life in organisms apparently bearing no close phylogenetic links but showing adaptations to perform the same functions are described as analogous, for example:
- Wings of bats, birds and insects;
- the jointed legs of insects and vertebrates;
- tail fin of fish, whale and lobster;
- eyes of the vertebrates and cephalopod molluscs (squid and octopus). Fig. 6 illustrates difference between an inverted and non-inverted retina, the sensory cells lying beneath the nerve fibres. This results in the sensory cells being absent where the optic nerve is attached to the eye, thus creating a blind spot. The octopus eye has a non-inverted retina in which the sensory cells lie above the nerve fibres. There is therefore no blind spot in this kind of eye. Apart from this difference the two eyes are remarkably similar, an example of convergent evolution.
Vestigial organs
Main article: Vestigial structure
A further aspect of comparative anatomy is the presence of vestigial organs. Organs that are smaller and simpler in structure than corresponding parts in the ancestral species are called vestigial organs. They are usually degenerated or underdeveloped. The existence of vestigial organs can be explained in terms of changes in the environment or modes of life of the species. Those organs are thought to be functional in the ancestral species but have now become unnecessary and non-functional. Examples are the vestigial hind limbs of whales, the haltere (vestigial hind wings) of flies and mosquitos, vestigial wings of flightless birds such as ostriches, and the vestigial leaves of some xerophytes (e.g. cactus) and parasitic plants (e.g. dodder). It must be noted however, that vestigial structures have lost the original function but may have another one. For example the halteres in dipterists help balance the insect while in flight and the wings of ostriches are used in mating rituals.
Evidence from geographical distribution
Biologists have discovered many puzzling facts about the presence of certain species on various continents and islands (biogeography).
Continental distribution
All organisms are adapted to their environment to a greater or lesser extent. If the abiotic and biotic factors within a habitat are capable of supporting a particular species in one geographic area, then one might assume that the same species would be found in a similar habitat in a similar geographic area, e.g. in Africa and South America. This is not the case. Plant and animal species are discontinuously distributed throughout the world:
- Africa has short-tailed (Old World) monkeys, elephants, lions and giraffes.
- South America has long-tailed monkeys, cougars, jaguars and llamas.
Even greater differences can be found if Australia is taken into consideration though it occupies the same latitude as South America and Africa. Marsupials like the kangaroo can be found in Australia, but are totally absent from Africa and are only represented by the opossum in South America and the Virginia Opossum in North America:
- The echidna and platypus, the only living representatives of primitive egg-laying mammals (monotremes), can be found only in Australia and are totally absent in the rest of the world.
- On the other hand, Australia has very few placental mammals except those that have been introduced by human beings.
Explanation
The main groups of modern mammal arose in Northern Hemisphere and subsequently migrated to three major directions:
- to South America via the land bridge in the Bering Strait and Isthmus of Panama; A large number of families of South American marsupials became extinct as a result of competition with these North American counterparts.
- to Africa via the Strait of Gibraltar; and
- to Australia via South East Asia to which it was at one time connected by land
The shallowness of the Bering Strait would have made the passage of animals between two northern continents a relatively easy matter, and it explains the present-day similarity of the two faunas. But once they had got down into the southern continents, they presumably became isolated from each other by various types of barriers.
- The submerging of the Isthmus of Panama: isolates the South American fauna
- the Mediterranean Sea and the North African desert: partially isolate the African fauna; and
- the submerging of the original connection between Australia and South East Asia: isolates the Australian fauna
Once isolated, the animals in each continent have shown adaptive radiation (Fig. 7) to evolve along their own lines.
Evidence for migration and isolation
The fossil record for the camel indicated that evolution of camels started in North America, from which they migrated across the Bering Strait into Asia and hence to Africa, and through the Isthmus of Panama into South America. Once isolated, they evolved along their own lines, giving the modern camel in Asia and Africa and llama in South America.
Continental drift
The same kinds of fossils are found from areas known to be adjacent to one another in the past but which, through the process of continental drift, are now in widely divergent geographic locations. For example, fossils of the same types of ancient amphibians, arthropods and ferns are found in South America, Africa, India, Australia and Antarctica, which can be dated to the Paleozoic Era, at which time these regions were united as a single landmass called Gondwana. [8] Sometimes the descendants of these organisms can be identified and show unmistakable similarity to each other, even though they now inhabit very different regions and climates.
Oceanic island distribution
Most small isolated islands only have native species that could have arrived by air or water; like birds, insects and turtles. The few large mammals present today were brought by human settlers in boats. Plant life on remote and recent volcanic islands like Hawaii could have arrived as airborne spores or as seeds in the droppings of birds. After the explosion of Krakatoa a century ago and the emergence of a steaming, lifeless remnant island called Anak Krakatoa (child of Krakatoa), plants arrived within months and within a year there were moths and spiders that had arrived by air. The island is now ecologially hard to distinguish from those around it that have been there for millions of years.
Evidence from comparative physiology and biochemistry
- See also: Archaeogenetics, Common descent, Last universal ancestor, Most recent common ancestor, Nothing in Biology Makes Sense Except in the Light of Evolution, Speciation, Timeline of evolution, Timeline of human evolution, Universal Code (Biology)
Evolution of widely distributed proteins and molecules
All known extant organisms make use of DNA and/or RNA. ATP is used as metabolic currency by all extant life. The Genetic code is the same for almost every organism, meaning that a piece of RNA in a bacterium codes for the same protein as in a human cell.
A classic example of biochemical evidence for evolution is the variance of the protein Cytochrome c in living cells. The variance of cytochrome c of different organisms is measured in the number of differing amino acids, each differing amino acid being a result of a base pair substitution, a mutation. If each differing amino acid is assumed to be the result of one base pair substitution, it can be calculated how long ago the two species diverged by multiplying the number of base pair substitutions by the estimated time it takes for a substituted base pair of the cytochrome c gene to be successfully passed on. For example, if the average time it takes for a base pair of the cytochrome c gene to mutate is N years, the number of amino acids making up the cytochrome c protein in monkeys differ by one from that of humans, this leads to the conclusion that the two species diverged N years ago.
Comparison of the DNA sequences allows organisms to be grouped by sequence similarity, and the resulting phylogenetic trees are typically congruent with traditional taxonomy, and are often used to strengthen or correct taxonomic classifications. Sequence comparison is considered a measure robust enough to be used to correct erroneous assumptions in the phylogenetic tree in instances where other evidence is scarce. For example, neutral human DNA sequences are approximately 1.2% divergent (based on substitutions) from those of their nearest genetic relative, the chimpanzee, 1.6% from gorillas, and 6.6% from baboons.[1] Genetic sequence evidence thus allows inference and quantification of genetic relatedness between humans and other apes.[2][3] The sequence of the 16S rRNA gene, a vital gene encoding a part of the ribosome, was used to find the broad phylogenetic relationships between all extant life. The analysis, originally done by Carl Woese, resulted in the three-domain system, arguing for two major splits in the early evolution of life. The first split led to modern Bacteria and the subsequent split led to modern Archaea and Eukaryote.
The proteomic evidence also supports the universal ancestry of life. Vital proteins, such as the ribosome, DNA polymerase, and RNA polymerase, are found in everything from the most primitive bacteria to the most complex mammals. The core part of the protein is conserved across all lineages of life, serving similar functions. Higher organisms have evolved additional protein subunits, largely affecting the regulation and protein-protein interaction of the core. Other overarching similarities between all lineages of extant organisms, such as DNA, RNA, amino acids, and the lipid bilayer, give support to the theory of common descent. The chirality of DNA, RNA, and amino acids is conserved across all known life. As there is no functional advantage to right- or left-handed molecular chirality, the simplest hypothesis is that the choice was made randomly by early organisms and passed on to all extant life through common descent. Further evidence for reconstructing ancestral lineages comes from junk DNA such as pseudogenes, "dead" genes which steadily accumulate mutations.[4]
There is also a large body of molecular evidence for a number of different mechanisms for large evolutionary changes, among them: genome and gene duplication, which facilitates rapid evolution by providing substantial quantities of genetic material under weak or no selective constraints; horizontal gene transfer, the process of transferring genetic material to another cell that is not an organism's offspring, allowing for species to acquire beneficial genes from each other; and recombination, capable of reassorting large numbers of different alleles and of establishing reproductive isolation. The Endosymbiotic theory explains the origin of mitochondria and plastids (e.g. chloroplasts), which are organelles of eukaryotic cells, as the incorporation of an ancient prokaryotic cell into ancient eukaryotic cell. Rather than evolving eukaryotic organelles slowly, this theory offers a mechanism for a sudden evolutionary leap by incorporating the genetic material and biochemical composition of a separate species. Evidence supporting this mechanism has recently been found in the protist Hatena: as a predator it engufes a green algae cell, which subsequently behaves as an endosymbiont, nourishing Hatena, which in turn loses it's feeding apparatus and behaves as an autotroph.[5][6]
Since metabolic processes do not leave fossils, research into the evolution of the basic cellular processes is done largely by comparison of existing organisms. Many lineages diverged when new metabolic processes appeared, and it is theoretically possible to determine when certain metabolic processes appeared by comparing the traits of the descendants of a common ancestor or by detecting their physical manifestations. As an example, the appearance of oxygen in the earth's atmosphere is linked to the evolution of photosynthesis.
Out of Africa hypothesis of human evolution
Mathematical models of evolution, pioneered by the likes of Sewall Wright, Ronald Fisher and J. B. S. Haldane and extended via diffusion theory by Motoo Kimura, allow predictions about the genetic structure of evolving populations. Direct examination of the genetic structure of modern populations via DNA sequencing has recently allowed verification of many of these predictions. For example, the Out of Africa theory of human origins, which states that modern humans developed in Africa and a small sub-population migrated out (undergoing a population bottleneck), implies that modern populations should show the signatures of this migration pattern. Specifically, post-bottleneck populations (Europeans and Asians) should show lower overall genetic diversity and a more uniform distribution of allele frequencies compared to the African population. Both of these predictions are borne out by actual data from a number of studies.[citation needed]
External link
Evidence from antibiotic and pesticide resistance
The development and spread of antibiotic resistant bacteria, like the spread of pesticide resistant forms of plants and insects is evidence for evolution of species, and of change within species. Thus the appearance of vancomycin resistant Staphlococcus aureus, and the danger it poses to hospital patients is a direct result of evolution through natural selection. Similarly the appearance of DDT resistance in various forms of Anopheles mosqitoes, and the appearance of myxomatosis resistance in breeding rabbit populations in Australia, are all evidence of the existence of evolution in situations of evolutionary selection pressure in species in which generations occur rapidly.
Evidence from studies of complex iteration
"It has taken more than five decades, but the electronic computer is now powerful enough to simulate evolution" [9] assisting bioinformatics in its attempt to solve biological problems.
Computer science allows the iteration of self changing complex systems to be studied, allowing a mathematically exact understanding of the nature of the processes behind evolution; providing evidence for the hidden causes of known evolutionary events. The evolution of specific cellular mechanisms like spliceosomes that can turn the cell's genome into a vast workshop of billions of interchangeable parts that can create tools that create tools that create tools that create us can be studied for the first time in an exact way.
For example, Christoph Adami et al. make this point in Evolution of biological complexity:
- To make a case for or against a trend in the evolution of complexity in biological evolution, complexity needs to be both rigorously defined and measurable. A recent information-theoretic (but intuitively evident) definition identifies genomic complexity with the amount of information a sequence stores about its environment. We investigate the evolution of genomic complexity in populations of digital organisms and monitor in detail the evolutionary transitions that increase complexity. We show that, because natural selection forces genomes to behave as a natural "Maxwell Demon," within a fixed environment, genomic complexity is forced to increase. [10]
For example, David J. Earl and Michael W. Deem make this point in Evolvability is a selectable trait:
- Not only has life evolved, but life has evolved to evolve. That is, correlations within protein structure have evolved, and mechanisms to manipulate these correlations have evolved in tandem. The rates at which the various events within the hierarchy of evolutionary moves occur are not random or arbitrary but are selected by Darwinian evolution. Sensibly, rapid or extreme environmental change leads to selection for greater evolvability. This selection is not forbidden by causality and is strongest on the largest-scale moves within the mutational hierarchy. Many observations within evolutionary biology, heretofore considered evolutionary happenstance or accidents, are explained by selection for evolvability. For example, the vertebrate immune system shows that the variable environment of antigens has provided selective pressure for the use of adaptable codons and low-fidelity polymerases during somatic hypermutation. A similar driving force for biased codon usage as a result of productively high mutation rates is observed in the hemagglutinin protein of influenza A. [11]
"Computer simulations of the evolution of linear sequences have demonstrated the importance of recombination of blocks of sequence rather than point mutagenesis alone. Repeated cycles of point mutagenesis, recombination, and selection should allow in vitro molecular evolution of complex sequences, such as proteins." [12] Evolutionary molecular engineering, also called directed evolution or in vitro molecular evolution involves the iterated cycle of mutation, multiplication with recombination, and selection of the fittest of individual molecules (proteins, DNA, and RNA). Natural evolution can be relived showing us possible paths from catalytic cycles based on proteins to based on RNA to based on DNA. [13] [14] [15] [16]
Evidence from speciation
Hawthorn fly
One example of evolution at work is the case of the hawthorn fly, Rhagoletis pomonella, also known as the apple maggot fly, which appears to be undergoing sympatric speciation.[7] Different populations of hawthorn fly feed on different fruits. A distinct population emerged in North America in the 19th century some time after apples, a non-native species, were introduced. This apple-feeding population normally feeds only on apples and not on the historically preferred fruit of hawthorns. The current hawthorn feeding population does not normally feed on apples. Some evidence, such as the fact that six out of thirteen allozyme loci are different, that hawthorn flies mature later in the season and take longer to mature than apple flies; and that there is little evidence of interbreeding (researchers have documented a 4-6% hybridization rate) suggests that this is occurring. The emergence of the new hawthorn fly is an example of evolution in progress.[8]
See also
- Human history
- Objections to evolution
ReferencesISBN links support NWE through referral fees
- ↑ Two sources: 'Genomic divergences between humans and other hominoids and the effective population size of the common ancestor of humans and chimpanzees'. and 'Quantitative Estimates of Sequence Divergence for Comparative Analyses of Mammalian Genomes' "[1] [2]"
- ↑ The picture labeled "Human Chromosome 2 and its analogs in the apes" in the article Comparison of the Human and Great Ape Chromosomes as Evidence for Common Ancestry is literally a picture of a link in humans that links two separate chromosomes in the nonhuman apes creating a single chromosome in humans. It is considered a missing link, and the ape-human connection is of particular interest. Also, while the term originally referred to fossil evidence, this too is a trace from the past corresponding to some living beings which when alive were the physical embodiment of this link.
- ↑ The New York Times report Still Evolving, Human Genes Tell New Story, based on A Map of Recent Positive Selection in the Human Genome, states the International HapMap Project is "providing the strongest evidence yet that humans are still evolving" and details some of that evidence.
- ↑ Pseudogene evolution and natural selection for a compact genome. "[3]"
- ↑ Okamoto N, Inouye I. (2005). A secondary symbiosis in progress. Science 310 (5746): 287.
- ↑ Okamoto N, Inouye I. (2006). Hatena arenicola gen. et sp. nov., a Katablepharid Undergoing Probable Plastid Acquisition.. Protist Article in Print.
- ↑ Feder et al (2003). Evidence for inversion polymorphism related to sympatric host race formation in the apple maggot fly, Rhagoletis pomonella.. Genetics 163 (3): 939-953.
- ↑ Berlocher, S.H. and G.L. Bush. 1982. An electrophoretic analysis of Rhagoletis (Diptera: Tephritidae) phylogeny. Systematic Zoology 31:136-155; Berlocher, S.H. and J.L. Feder. 2002. Sympatric speciation in phytophagous insects: moving beyond controversy? Annual Review of Entomology 47:773-815; Bush, G.L. 1969. Sympatric host race formation and speciation in frugivorous flies of the genus Rhagoletis (Diptera: Tephritidae). Evolution 23:237-251; Prokopy, R.J., S.R. Diehl and S.S. Cooley. 1988. Behavioral evidence for host races in Rhagoletis pomonella flies. Oecologia 76:138-147. Proc. Natl. Acad. Sci. USA - Vol. 94, pp. 11417-11421, October 1997 - Evolution article Selective maintenance of allozyme differences among sympatric host races of the apple maggot fly.
- Darwin, Charles November 24 1859. On the Origin of Species by means of Natural Selection or the Preservation of Favoured Races in the Struggle for Life. London: John Murray, Albemarle Street. 502 pages. Reprinted: Gramercy (May 22, 1995). ISBN 0-517-12320-7
- Mayr, Ernst. What Evolution Is. Basic Books (October, 2002). ISBN 0-465-04426-3
- Gigerenzer, Gerd, et al., The empire of chance: how probability changed science and everyday life (New York: Cambridge University Press, 1989).
- Williams, G.C. (1966). Adaptation and Natural Selection: A Critique of some Current Evolutionary Thought. Princeton, N.J.: Princeton University Press.
- Biological science, Oxford, 2002.
- CJ Clegg, 1999, Genetics and Evolution, John Murray. ISBN 0-7195-7552-4
- Y.K. Ho, 2004, Advanced-level Biology for Hong Kong, Manhattan Press. ISBN 962-990-635-X
- Paul, Christopher R. C. (1998) The Adequacy of the Fossil Record, John Wiley & Sons, ISBN 0-471-96988-5
- Behrensmeyer, Anna K. (1980) Fossils in the making: Vertebrate taphonomy and paleoecology, University of Chicago Press, ISBN 0-226-04169-7
- Martin, Ronald E. et al. eds. (1999) Taphonomy: A Process Approach, Cambridge University Press, ISBN 0-521-59833-8
External links
- 29+ Evidences for Macroevolution: The Scientific Case for Common Descent
- A critique of the aforementioned 29+ Evidences for Macroevolution
- Response to the "critique"
- National Academies Evolution Resources
- Evolution by Natural Selection — An introduction to the logic of the theory of evolution by natural selection
- Evolution — Provided by PBS.
- Howstuffworks.com — How Evolution Works
- Evolution News from Genome News Network (GNN)
- National Academy Press: Teaching About Evolution and the Nature of Science
- Transitional Vertebrate Fossils FAQ
Basic topics in evolutionary biology | (edit) |
---|---|
Processes of evolution: evidence - macroevolution - microevolution - speciation | |
Mechanisms: natural selection - genetic drift - gene flow - mutation - phenotypic plasticity | |
Modes: anagenesis - catagenesis - cladogenesis | |
History: History of evolutionary thought - Charles Darwin - The Origin of Species - modern evolutionary synthesis | |
Subfields: population genetics - ecological genetics - human evolution - molecular evolution - phylogenetics - systematics |
Credits
New World Encyclopedia writers and editors rewrote and completed the Wikipedia article in accordance with New World Encyclopedia standards. This article abides by terms of the Creative Commons CC-by-sa 3.0 License (CC-by-sa), which may be used and disseminated with proper attribution. Credit is due under the terms of this license that can reference both the New World Encyclopedia contributors and the selfless volunteer contributors of the Wikimedia Foundation. To cite this article click here for a list of acceptable citing formats.The history of earlier contributions by wikipedians is accessible to researchers here:
The history of this article since it was imported to New World Encyclopedia:
Note: Some restrictions may apply to use of individual images which are separately licensed.