Difference between revisions of "Coenzyme" - New World Encyclopedia
Rick Swarts (talk | contribs) |
|||
(37 intermediate revisions by 3 users not shown) | |||
Line 1: | Line 1: | ||
− | + | {{Images OK}}{{Approved}}{{copyedited}} | |
− | |||
− | + | [[Image:NAD oxidation reduction.svg|thumb|right|250px|The [[redox]] reactions of [[nicotinamide adenine dinucleotide]].]] | |
− | + | '''Coenzyme''' is any of a diverse group of small [[organic compound|organic]], non-[[protein]], freely diffusing [[molecule]]s that are loosely associated with and essential for the activity of [[enzyme]]s, serving as carrier molecules that transfer chemical groups. Coenzymes are sometimes referred to as ''cosubstrates.'' These molecules are [[Substrate (biochemistry) |substrate]]s for enzymes and do not form a permanent part of the enzymes' structures. | |
+ | The term coenzymes is sometimes defined in such a way as to include [[prosthetic group]]s (Alberts et al. 1989; Bender and Bender 2005; McGraw-Hill 2005). However, prosthetic groups are non-protein components that are ''bound tightly'' (covalently linked) to enzymes—such as [[iron-sulfur protein|iron-sulfur centers]], [[flavin]], or [[haem]] groups. The International Union of Pure and Applied Chemistry (IUPAC) draws a distinction between coenzymes and prosthetic groups. IUPAC defines a coenzyme as a low-molecular-weight, non-protein organic compound that is ''loosely attached,'' participating in enzymatic reactions as a dissociable acceptor of chemical groups or electrons; a prosthetic group is defined as a ''tightly bound,'' nonpolypeptide unit in a protein (IUPAC 1997a, 1997b). Both coenzymes and prosthetic groups are types of the broader group of [[cofactor (biochemistry)|cofactors]], which are any non-protein molecules (usually organic molecules or metal ions) that are required by an enzyme for its activity (IUPAC 1997c). This article will restrict coenzyme to the definition used by IUPAC. | ||
− | + | Well-known coenzymes include [[adenosine triphosphate]] (ATP), which transfers phosphate groups; [[nicotinamide adenine dinucleotide]] (NADH, NADPH), which transfers hydrogens and electrons; coenzyme A, which transfers acetyl groups; and S-adenosylmethionine, which transfers methyl groups (Alberts et al. 1989). | |
+ | In [[metabolism]], coenzymes are involved in both group-transfer reactions, for example [[coenzyme A]] and [[ATP]], and redox reactions, such as [[Coenzyme Q10|coenzyme Q<sub>10</sub>]] and NAD<sup>+</sup>. Coenzymes are consumed and recycled continuously in metabolism, with one set of enzymes adding a chemical group to the coenzyme and another set removing it. For example, enzymes such as [[ATP synthase]] continuously [[phosphorylation|phosphorylate]] [[adenosine diphosphate]] (ADP), converting it into ATP, while enzymes such as [[kinase]]s dephosphorylate the ATP and convert it back to ADP. | ||
+ | {{toc}} | ||
+ | Coenzymes molecules are often [[vitamin]]s or are made from vitamins. Many coenzymes contain the [[nucleotide]] [[adenosine]] as part of their structures, such as ATP, coenzyme A, and [[NAD%2B|NAD<sup>+</sup>]]. | ||
− | + | Coenzymes are vastly important in life. Some, such as ATP and NADH, form a core part of [[metablolism]] and reflect the unity in nature, being present in all known forms of [[life]]. | |
− | + | ==Coenzymes as metabolic intermediates== | |
− | + | [[Metabolism]] involves a vast array of chemical reactions, but most fall under a few basic types of reactions that involve the transfer of [[functional group]]s (Mitchell 1979). This common chemistry allows cells to use a small set of metabolic intermediates to carry chemical groups between different reactions (Wimmer and Rose 1978). These group-transfer intermediates are the coenzymes. | |
− | + | Each class of group-transfer reaction is carried out by a particular coenzyme, which is the substrate for a set of [[enzyme]]s that produce it, and a set of enzymes that consume it. An example of this are the [[dehydrogenase]]s that use [[nicotinamide adenine dinucleotide]] (NADH) as a cofactor. Here, hundreds of separate types of enzymes remove electrons from their substrates and [[redox|reduce]] NAD<sup>+</sup> to NADH. This reduced coenzyme is then a substrate for any of the [[reductase]]s in the cell that need to reduce their substrates (Pollak et al. 2007). | |
− | + | Coenzymes are therefore continuously recycled as part of metabolism. As an example, the total quantity of ATP in the human body is about 0.1 [[Mole (unit)|mole]]. This ATP is constantly being broken down into ADP, and then converted back into ATP. Thus, at any given time, the total amount of ATP + ADP remains fairly constant. The energy used by human cells requires the [[hydrolysis]] of 100 to 150 moles of ATP daily, which is around 50 to 75 kilograms. Typically, a human will use up their body weight of ATP over the course of the day (Di Carlo and Collins 2001). This means that each ATP molecule is recycled 1000 to 1500 times daily. | |
− | |||
− | |||
− | |||
− | |||
− | |||
− | |||
− | |||
− | Coenzymes are therefore continuously recycled as part of metabolism. As an example, the total quantity of ATP in the human body is about 0.1 [[Mole (unit)|mole]]. This ATP is constantly being broken down into ADP, and then converted back into ATP. Thus, at any given time, the total amount of ATP + ADP remains fairly constant. The energy used by human cells requires the [[hydrolysis]] of 100 to 150 moles of ATP daily which is around 50 to 75 | ||
==Types== | ==Types== | ||
− | Acting as coenzymes in organisms is the major role of [[vitamin]]s, although vitamins do have other functions in the body | + | Acting as coenzymes in organisms is the major role of [[vitamin]]s, although vitamins do have other functions in the body (Bolander 2006). Coenzymes are also commonly made from [[nucleotide]]s, such as [[adenosine triphosphate]], the biochemical carrier of [[phosphate]] groups, or [[coenzyme A]], the coenzyme that carries [[acyl]] groups. Most coenzymes are found in a huge variety of species, and some are universal to all forms of life. An exception to this wide distribution is a group of unique coenzymes that evolved in [[methanogen]]s, which are restricted to this group of [[archaea]] (Rouvière and Wolfe 1988). |
===Vitamins and derivatives=== | ===Vitamins and derivatives=== | ||
Line 35: | Line 30: | ||
| '''Coenzyme''' || '''Vitamin''' || '''Additional component''' || '''Chemical group(s) transferred''' || '''Distribution''' | | '''Coenzyme''' || '''Vitamin''' || '''Additional component''' || '''Chemical group(s) transferred''' || '''Distribution''' | ||
|- | |- | ||
− | | [[Nicotinamide adenine dinucleotide|NAD<sup>+</sup>]] and [[Nicotinamide adenine dinucleotide phosphate|NADP<sup>+</sup>]] <ref | + | | [[Nicotinamide adenine dinucleotide|NAD<sup>+</sup>]] and [[Nicotinamide adenine dinucleotide phosphate|NADP<sup>+</sup>]] <ref>Pollak et al. (2007)</ref> || [[Niacin]] (B<sub>3</sub>) || ADP || [[Electron]]s || [[Bacteria]], [[archaea]], and [[eukaryote]]s |
|- | |- | ||
− | | [[Coenzyme A]] <ref> | + | | [[Coenzyme A]] <ref>Leonardi et al. (2005).</ref> || [[Pantothenic acid]] (B<sub>5</sub>) || ADP || [[Acetyl|Acetyl group]] and other [[acyl|acyl groups]] || [[Bacteria]], [[archaea]] and [[eukaryote]]s |
|- | |- | ||
− | | [[Tetrahydrofolic acid]] <ref> | + | | [[Tetrahydrofolic acid]] <ref>Donnelly (2001) </ref> || [[Folic acid]] (B<sub>9</sub>) || [[glutamic acid|Glutamate]] residues || [[Methyl]], [[aldehyde|formyl]], [[methylene]] and formimino groups || [[Bacteria]], [[archaea]] and [[eukaryote]]s |
|- | |- | ||
− | |[[Vitamin K|Menaquinone]] <ref | + | |[[Vitamin K|Menaquinone]] <ref>Søballe and Poole (1999).</ref> || Vitamin K || None || [[Carbonyl|Carbonyl group]] and [[electron]]s || [[Bacteria]], [[archaea]] and [[eukaryote]]s |
|- | |- | ||
− | |[[Ascorbic acid]] <ref> | + | |[[Ascorbic acid]] <ref>Linster and Van Schaftingen (2007)</ref> || Vitamin C || None || [[Electron]]s || [[Bacteria]], [[archaea]] and [[eukaryote]]s |
|- | |- | ||
− | |[[Coenzyme F420]] <ref> | + | |[[Coenzyme F420]] <ref>Mack and Grill (2006).</ref> || [[Riboflavin]] (B<sub>2</sub>) || Amino acids || [[Electron]]s || [[Methanogen]]s and some [[bacteria]] |
|- | |- | ||
|} | |} | ||
Line 53: | Line 48: | ||
| '''Coenzyme''' || '''Chemical group(s) transferred''' || '''Distribution''' | | '''Coenzyme''' || '''Chemical group(s) transferred''' || '''Distribution''' | ||
|- | |- | ||
− | |[[Adenosine triphosphate]] <ref> | + | |[[Adenosine triphosphate]] <ref>Knowles (1980).</ref> || [[Phosphate|Phosphate group]] || [[Bacteria]], [[archaea]] and [[eukaryote]]s |
|- | |- | ||
− | |[[S-Adenosyl methionine]] <ref> | + | |[[S-Adenosyl methionine]] <ref>Chiang et al. (1996).</ref> || [[Methyl group]] || [[Bacteria]], [[archaea]] and [[eukaryote]]s |
|- | |- | ||
− | |[[3'-Phosphoadenosine-5'-phosphosulfate]] <ref> | + | |[[3'-Phosphoadenosine-5'-phosphosulfate]] <ref>Negishi et al. (2001).</ref> || [[Sulfate|Sulfate group]] || [[Bacteria]], [[archaea]] and [[eukaryote]]s |
|- | |- | ||
− | | [[Coenzyme Q]] <ref> | + | | [[Coenzyme Q]] <ref>Crane (2001)</ref> || [[Electron]]s || [[Bacteria]], [[archaea]], and [[eukaryote]]s |
|- | |- | ||
− | |[[Tetrahydrobiopterin]] <ref> | + | |[[Tetrahydrobiopterin]] <ref>Thony et al. (2000).</ref> || [[Oxygen]] atom and [[electron]]s || [[Bacteria]], [[archaea]], and [[eukaryote]]s |
|- | |- | ||
− | |[[Cytidine triphosphate]] <ref | + | |[[Cytidine triphosphate]] <ref>Buchanan et al. (2000)</ref>|| [[Diacylglycerol]]s and lipid head groups || [[Bacteria]], [[archaea]], and [[eukaryote]]s |
|- | |- | ||
− | |[[Nucleotide sugar]]s <ref | + | |[[Nucleotide sugar]]s <ref>Ginsburg (1978).</ref>|| [[Monosaccharide]]s || [[Bacteria]], [[archaea]], and [[eukaryote]]s |
|- | |- | ||
− | |[[Glutathione]] <ref> | + | |[[Glutathione]] <ref>Grill et al. (2001); Meister and Anderson (1983).</ref>|| [[Electron]]s || Some [[bacteria]] and most [[eukaryote]]s |
|- | |- | ||
− | | [[Coenzyme M]] <ref> | + | | [[Coenzyme M]] <ref>Taylor and Wolfe (1974); Balch and Wolfe (1979).</ref> || [[Methyl group]] || [[Methanogen]]s |
|- | |- | ||
− | |[[Coenzyme B]] <ref> | + | |[[Coenzyme B]] <ref>Noll et al. 1986).</ref> || [[Electron]]s || [[Methanogen]]s |
|- | |- | ||
− | |[[Methanofuran]] <ref> | + | |[[Methanofuran]] <ref>Vorholt and Thauer (1997).</ref> || [[aldehyde|Formyl group]] || [[Methanogen]]s |
|- | |- | ||
− | |[[Tetrahydromethanopterin]] <ref> | + | |[[Tetrahydromethanopterin]] <ref>DiMarco et al. (1990).</ref> || [[Methyl group]] || [[Methanogen]]s |
|} | |} | ||
− | == | + | ==History== |
− | + | The first coenzyme to be discovered was NAD<sup>+</sup>, which was identified by [[Arthur Harden]] and William Youndin and reported on in 1906 (Harden and Young 1906). They noticed that adding boiled and filtered [[yeast]] extract greatly accelerated [[alcoholic fermentation]] in unboiled yeast extracts. They called the unidentified factor responsible for this effect a ''coferment''. Through a long and difficult purification from yeast extracts, this heat-stable factor was identified as a [[nucleotide]] sugar phosphate by [[Hans von Euler-Chelpin]] (1930). Other coenzymes were identified throughout the early twentieth century, with ATP being isolated in 1929, by Karl Lohmann (1929), and coenzyme A being discovered in 1945, by [[Fritz Albert Lipmann]] (1945). | |
− | |||
− | + | The functions of coenzymes were at first mysterious, but in 1936, [[Otto Heinrich Warburg]] identified the function of NAD<sup>+</sup> in hydride transfer (Warburg and Christian (1936). This discovery was followed in the early 1940s by the work of [[Herman Kalckar]], who established the link between the oxidation of sugars and the generation of ATP (Kalckar 1974). This confirmed the central role of ATP in energy transfer that had been proposed by Fritz Albert Lipmann in 1941 (Lipmann (1941). Later, in 1949, Morris Friedkin and [[Albert L. Lehninger]] proved that the coenzyme NAD<sup>+</sup> linked metabolic pathways, such as the citric acid cycle and the synthesis of ATP (Friedkin and Lehninger 1949). | |
− | == | + | ==Evolution== |
− | + | Coenzymes, such as [[Adenosine triphosphate|ATP]] and [[NADH]], are present in all known forms of [[life]] and form a core part of [[metabolism]]. Such universal [[Conservation (genetics)|conservation]] indicates that these molecules evolved very early in the development of living things (Chen et al. 2007). At least some of the current set of coenzymes may therefore have been present in the [[last universal ancestor]], which lived about 4 billion years ago (Koch 1998; Ouzounis and Kyrpides 1996). | |
− | |||
− | + | Coenzymes may have been present even earlier in the [[Timeline of evolution|history of life]] on Earth (White 1976). Interestingly, the nucleotide [[adenosine]] is present in coenzymes that catalyse many basic metabolic reactions such as methyl, acyl, and phosphoryl group transfer, as well as [[redox]] reactions. This ubiquitous chemical scaffold has therefore been proposed to be a remnant of the [[RNA world hypothesis|RNA world]], with early [[ribozyme]]s evolving to bind a restricted set of nucleotides and related compounds (Saran et al. 2003; Jadhav and Yarus 2002). Adenosine-based coenzymes are thought to have acted as interchangeable adaptors that allowed enzymes and ribozymes to bind new coenzymes through small modifications in existing adenosine-binding [[protein domain|domain]]s, which had originally evolved to bind a different cofactor (Denessiouk et al. 2001). This process of adapting a pre-evolved structure for a novel use is referred to as ''[[exaptation]]''. | |
− | == | + | ==Notes== |
− | + | <references/> | |
− | |||
− | |||
==References== | ==References== | ||
− | + | * Alberts, B., D. Bray, J. Lewis, M. Raff, K. Roberts, and J.D. Watson. ''Molecular Biology of the Cell,'' 2nd edition. New York: Garland Publishing, 1989. ISBN 0824036956. | |
− | + | * Balch, W.E., and R.S. Wolfe. 1979. [http://www.pubmedcentral.nih.gov/picrender.fcgi?artid=218444&blobtype=pdf Specificity and biological distribution of coenzyme M (2-mercaptoethanesulfonic acid)]. ''J. Bacteriol.'' 137(1): 256–63. PMID 104960. Retrieved August 12, 2008. | |
− | + | * Bender, D.A., and A.E. Bender. 2005. ''A Dictionary of Food and Nutrition''. New York: Oxford University Press. ISBN 0198609612. | |
− | + | * Biocarta [http://www.biocarta.com/pathfiles/m_g1pPathway.asp Leloir pathway of galactose metabolism.] Charting pathways of life. Retrieved August 4, 2008. | |
− | McGraw Hill | + | * Bolander, F.F. 2006. [http://www.ncbi.nlm.nih.gov/pubmed/17086936 Vitamins: Not just for enzymes.] ''Curr Opin Investig Drugs'' 7(10): 912–5. PMID 17086936. |
+ | * Buchanan, B.B., W. Gruissem, and R.L. Jones. 2000. ''Biochemistry & Molecular Biology of Plants,'' 1st edition. American Society of Plant Physiology. ISBN 0943088399. | ||
+ | * Chen, X., N. Li, and A. D. Ellington. 2007. [http://www.ncbi.nlm.nih.gov/pubmed/17443876 Ribozyme catalysis of metabolism in the RNA world]. ''Chem. Biodivers.'' 4(4): 633–55. PMID 17443876. Retrieved August 12, 2008. | ||
+ | * Chiang, P., R. Gordon, J. Tal, G. Zeng, B. Doctor, and K. Pardhasaradhi, and P. McCann. 1996. [http://www.ncbi.nlm.nih.gov/pubmed/8647346 S-Adenosylmethionine and methylation.] ''FASEB J'' 10(4): 471–80. PMID 8647346. Retrieved August 12, 2008. | ||
+ | * Crane, F.L. 2001. [http://www.jacn.org/cgi/content/full/20/6/591 Biochemical functions of coenzyme Q10.] ''Journal of the American College of Nutrition'' 20(6): 591-8. PMID 11771674. Retrieved August 12, 2008. | ||
+ | * Denessiouk, K.A., V.V. Rantanen, and M.S. Johnson. 2001. [http://www.ncbi.nlm.nih.gov/pubmed/11455601 Adenine recognition: A motif present in ATP-, CoA-, NAD-, NADP-, and FAD-dependent proteins.] ''Proteins'' 44(3): 282–91. PMID 11455601. Retrieved August 12, 2008. | ||
+ | * Di Carlo, S.E., and H.L. Collins. 2001. [http://advan.physiology.org/cgi/content/full/25/2/70 Estimating ATP resynthesis during a marathon run: A method to introduce metabolism.] ''Advan. Physiol. Edu.'' 25: 70-71. Retrieved August 12, 2008. | ||
+ | * DiMarco, A. A., T. A. Bobik, and R. S. Wolfe. 1990. [http://www.ncbi.nlm.nih.gov/pubmed/2115763 Unusual coenzymes of methanogenesis.] ''Annu. Rev. Biochem.'' 59: 355–94. PMID 2115763. Retrieved August 12, 2008. | ||
+ | * Donnelly, J. G. 2001. [http://www.ncbi.nlm.nih.gov/pubmed/11451208 Folic acid]. ''Crit Rev Clin Lab Sci'' 38(3): 183–223. PMID 11451208. Retrieved August 12, 2008. | ||
+ | * Friedkin, M., and A.L. Lehninger. 1949. [http://www.jbc.org/cgi/reprint/178/2/611 Esterification of inorganic phosphate coupled to electron transport between dihydrodiphosphopyridine nucleotide and oxygen.] ''J. Biol. Chem.'' 178(2): 611–23. PMID 18116985. Retrieved August 12, 2008. | ||
+ | * Ginsburg, V. 1978. [http://www.ncbi.nlm.nih.gov/pubmed/351635 Comparative biochemistry of nucleotide-linked sugars.] ''Prog. Clin. Biol. Res.'' 23: 595–600. PMID 351635. | ||
+ | * Grill, D., T. Tausz, and L.J. De Kok. 2001. [http://books.google.com/books?hl=sv&lr=&id=aX2eJf1i67IC&oi=fnd&pg=PA13&ots=8feo-QOEPa&sig=XAMjZ0Wan17vmoUKg_FFNRl8g0I#PPP1,M1 Significance of glutathione in plant adaptation to the environment.] Springer. ISBN 1402001789. Retrieved August 12,2008. | ||
+ | * Harden, A., and W.J. Young. 1906. The alcoholic ferment of yeast-juice. ''Proceedings of the Royal Society of London. Series B, Containing Papers of a Biological Character'' 78(526): 369-375. | ||
+ | * International Union of Pure and Applied Chemistry (IUPAC), Inorganic Chemistry Division, Commission on Nomenclature of Inorganic Chemistry. 1997a. [http://www.chem.qmul.ac.uk/iupac/bioinorg/CD.html#33 Glossary of terms used in bioinorganic chemistry: '''Coenzyme.'''] From M. W. G. de Bolster, ''Pure Appl. Chem.'' 69: 1251-1303. Retrieved August 12, 2008. | ||
+ | * —. 1997b. [http://www.chem.qmul.ac.uk/iupac/bioinorg/PR.html#24 Glossary of terms used in bioinorganic chemistry: '''Prosthetic group.'''] From M. W. G. de Bolster, ''Pure Appl. Chem.'' 69: 1251-1303. Retrieved August 12, 2008. | ||
+ | * —. 1997c. [http://www.chem.qmul.ac.uk/iupac/bioinorg/CD.html#34 Glossary of terms used in bioinorganic chemistry: '''Cofactor.'''] From M. W. G. de Bolster, ''Pure Appl. Chem.'' 69: 1251-1303. Retrieved August 12, 2008. | ||
+ | * Jadhav, V. R., and M. Yarus. 2002. [http://www.ncbi.nlm.nih.gov/pubmed/12458080 Coenzymes as coribozymes.] ''Biochimie'' 84(9): 877–88. PMID 12458080. | ||
+ | * Kalckar, H. M. 1974. [http://www.ncbi.nlm.nih.gov/pubmed/4279328 Origins of the concept oxidative phosphorylation.] ''Mol. Cell. Biochem.'' 5(1–2): 55-63. PMID 4279328. Retrieved August 12, 2008. | ||
+ | * Knowles, J. R. 1980. [http://www.ncbi.nlm.nih.gov/pubmed/6250450 Enzyme-catalyzed phosphoryl transfer reactions.] ''Annu. Rev. Biochem.'' 49: 877–919. PMID 6250450. Retrieved August 12, 2008. | ||
+ | * Koch, A. 1998. [http://www.ncbi.nlm.nih.gov/pubmed/9889982 How did bacteria come to be?] ''Adv Microb Physiol'' 40: 353–99. PMID 9889982. Retrieved August 12, 2008. | ||
+ | * Leonardi, R., Y. M. Zhang, C. O. Rock, and S. Jackowski. 2005. [http://www.ncbi.nlm.nih.gov/pubmed/15893380 Coenzyme A: Back in action.] ''Prog. Lipid Res.'' 44(2-3): 125–53. PMID 15893380. Retrieved August 12, 2008. | ||
+ | * Linster, C. L., and E. Van Schaftingen. 2007. [http://www.ncbi.nlm.nih.gov/pubmed/17222174 Vitamin C. Biosynthesis, recycling and degradation in mammals.] ''FEBS J.'' 274(1): 1–22. PMID 17222174. Retrieved August 12, 2008. | ||
+ | * Lipmann, F. 1945. [http://www.jbc.org/cgi/reprint/160/1/173 Acetylation of sulfanilamide by liver homogenates and extracts.] ''J. Biol. Chem.'' 160(1): 173–190. Retrieved August 12, 2008. | ||
+ | * Lipmann, F. 1941. Metabolic generation and utilization of phosphate bond energy. ''Adv Enzymol'' 1: 99-162. | ||
+ | * Lohmann, K. 1929. Über die Pyrophosphatfraktion im Muskel. ''Naturwissenschaften'' 17: 624–625. | ||
+ | * Mack, M., and S. Grill. 2006. [http://www.ncbi.nlm.nih.gov/pubmed/16607521 Riboflavin analogs and inhibitors of riboflavin biosynthesis.] ''Appl. Microbiol. Biotechnol.'' 71(3): 265–75. PMID 16607521. Retrieved August 12, 2008. | ||
+ | * ''McGraw-Hill Concise Encyclopedia of Science & Technology''. 2005. New York: McGraw-Hill. ISBN 0071429573. | ||
+ | * Meister, A., and M. E. Anderson. 1983. [http://www.ncbi.nlm.nih.gov/pubmed/6137189 Glutathione.] ''Annu. Rev. Biochem.'' 52: 711–60. PMID 6137189. Retrieved August 12, 2008. | ||
+ | * Mitchell, P. 1979. [http://www.ncbi.nlm.nih.gov/pubmed/378655 The Ninth Sir Hans Krebs Lecture. Compartmentation and communication in living systems. Ligand conduction: a general catalytic principle in chemical, osmotic and chemiosmotic reaction systems.] ''Eur J Biochem'' 95(1): 1-20. PMID 378655. Retrieved August 12, 2008. | ||
+ | * Negishi, M., L.G. Pedersen, E. Petrotchenko, ''et al''. 2001. [http://www.ncbi.nlm.nih.gov/pubmed/11396917 Structure and function of sulfotransferases. ''Arch. Biochem. Biophys.]'' 390(2): 149–57. PMID 11396917. Retrieved August 12, 2008. | ||
+ | * Noll, K.M., K.L. Rinehart, R.S. Tanner, and R.S. Wolfe. 1986. [http://www.pubmedcentral.nih.gov/articlerender.fcgi?tool=pubmed&pubmedid=3086878 Structure of component B (7-mercaptoheptanoylthreonine phosphate) of the methylcoenzyme M methylreductase system of Methanobacterium thermoautotrophicum]. ''Proc. Natl. Acad. Sci. U.S.A.'' 83(12): 4238–42. PMID 3086878. Retrieved August 12, 2008. | ||
+ | * Ouzounis, C., and N. Kyrpides. 1996. [http://www.ncbi.nlm.nih.gov/pubmed/8706840 The emergence of major cellular processes in evolution.] ''FEBS Lett'' 390(2): 119-23. PMID 8706840. Retrieved August 12, 2008. | ||
+ | * Pollak, N., C. Dölle, and M. Ziegler. 2007. [http://www.pubmedcentral.nih.gov/articlerender.fcgi?tool=pubmed&pubmedid=17295611 The power to reduce: Pyridine nucleotides—small molecules with a multitude of functions.] ''Biochem. J.'' 402(2): 205-18. PMID 17295611. Retrieved August 12, 2008. | ||
+ | * Rouvière, P.E., and R.S. Wolfe. 1988. [http://www.jbc.org/cgi/reprint/263/17/7913 Novel biochemistry of methanogenesis.] ''J. Biol. Chem.'' 263(17): 7913–6. PMID 3131330. Retrieved August 12, 2008. | ||
+ | * Saran, D., J. Frank, and D. H. Burke. 2003. [http://www.pubmedcentral.nih.gov/articlerender.fcgi?tool=pubmed&pubmedid=14687414 The tyranny of adenosine recognition among RNA aptamers to coenzyme A.] ''BMC Evol. Biol.'' 3: 26. PMID 14687414. Retrieved August 12, 2008. | ||
+ | * Søballe, B., and R. K. Poole. 1999. [http://mic.sgmjournals.org/cgi/reprint/145/8/1817.pdf Microbial ubiquinones: Multiple roles in respiration, gene regulation and oxidative stress management.] ''Microbiology'' 145: 1817-30. PMID 10463148. Retrieved August 12, 2008. | ||
+ | * Taylor, C. D., and R. S. Wolfe. 1974. [http://www.jbc.org/cgi/reprint/249/15/4879 Structure and methylation of coenzyme M(HSCH2CH2SO3).] ''J. Biol. Chem.'' 249(15): 4879–85. PMID 4367810. Retrieved August 12, 2008. | ||
+ | * Thony, B., G. Auerbach, and N. Blau. 2000. [http://www.pubmedcentral.gov/articlerender.fcgi?tool=pubmed&pubmedid=10727395 Tetrahydrobiopterin biosynthesis, regeneration and functions.] ''Biochem J'' 347: 1–16. PMID 10727395. Retrieved August 12, 2008. | ||
+ | * von Euler-Chelpin, H. 1930. [http://nobelprize.org/nobel_prizes/chemistry/laureates/1929/euler-chelpin-lecture.pdf Fermentation of sugars and fermentative enzymes: Nobel Lecture, May 23, 1930.] ''Nobel Foundation''. Retrieved August 12, 2008. | ||
+ | * Vorholt, J. A., and R. K. Thauer. 1997. [http://www.ncbi.nlm.nih.gov/pubmed/9342247 The active species of 'CO2' utilized by formylmethanofuran dehydrogenase from methanogenic Archaea.] ''Eur. J. Biochem.'' 248(3): 919–24. PMID 9342247. Retrieved August 12, 2008. | ||
+ | * Warburg, O., and W. Christian. 1936. Pyridin, the hydrogen-transferring component of the fermentation enzymes (pyridine nucleotide). ''Biochemische Zeitschrift'' 287: 291. | ||
+ | * White, H. B. 1976. [http://www.ncbi.nlm.nih.gov/pubmed/1263263 Coenzymes as fossils of an earlier metabolic state.] ''J. Mol. Evol.'' 7(2): 101–4. PMID 1263263. Retrieved August 12, 2008. | ||
+ | * Wimmer, M., and I. Rose. 1978. [http://www.ncbi.nlm.nih.gov/pubmed/354490 Mechanisms of enzyme-catalyzed group transfer reactions.] ''Annu Rev Biochem'' 47: 1031-78. PMID 354490. Retrieved August 12, 2008. | ||
− | |||
− | |||
− | |||
− | |||
− | |||
− | |||
{{Enzyme cofactors}} | {{Enzyme cofactors}} | ||
[[Category:Life sciences]] | [[Category:Life sciences]] |
Latest revision as of 07:19, 6 June 2023
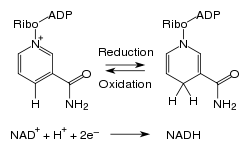
Coenzyme is any of a diverse group of small organic, non-protein, freely diffusing molecules that are loosely associated with and essential for the activity of enzymes, serving as carrier molecules that transfer chemical groups. Coenzymes are sometimes referred to as cosubstrates. These molecules are substrates for enzymes and do not form a permanent part of the enzymes' structures.
The term coenzymes is sometimes defined in such a way as to include prosthetic groups (Alberts et al. 1989; Bender and Bender 2005; McGraw-Hill 2005). However, prosthetic groups are non-protein components that are bound tightly (covalently linked) to enzymes—such as iron-sulfur centers, flavin, or haem groups. The International Union of Pure and Applied Chemistry (IUPAC) draws a distinction between coenzymes and prosthetic groups. IUPAC defines a coenzyme as a low-molecular-weight, non-protein organic compound that is loosely attached, participating in enzymatic reactions as a dissociable acceptor of chemical groups or electrons; a prosthetic group is defined as a tightly bound, nonpolypeptide unit in a protein (IUPAC 1997a, 1997b). Both coenzymes and prosthetic groups are types of the broader group of cofactors, which are any non-protein molecules (usually organic molecules or metal ions) that are required by an enzyme for its activity (IUPAC 1997c). This article will restrict coenzyme to the definition used by IUPAC.
Well-known coenzymes include adenosine triphosphate (ATP), which transfers phosphate groups; nicotinamide adenine dinucleotide (NADH, NADPH), which transfers hydrogens and electrons; coenzyme A, which transfers acetyl groups; and S-adenosylmethionine, which transfers methyl groups (Alberts et al. 1989).
In metabolism, coenzymes are involved in both group-transfer reactions, for example coenzyme A and ATP, and redox reactions, such as coenzyme Q10 and NAD+. Coenzymes are consumed and recycled continuously in metabolism, with one set of enzymes adding a chemical group to the coenzyme and another set removing it. For example, enzymes such as ATP synthase continuously phosphorylate adenosine diphosphate (ADP), converting it into ATP, while enzymes such as kinases dephosphorylate the ATP and convert it back to ADP.
Coenzymes molecules are often vitamins or are made from vitamins. Many coenzymes contain the nucleotide adenosine as part of their structures, such as ATP, coenzyme A, and NAD+.
Coenzymes are vastly important in life. Some, such as ATP and NADH, form a core part of metablolism and reflect the unity in nature, being present in all known forms of life.
Coenzymes as metabolic intermediates
Metabolism involves a vast array of chemical reactions, but most fall under a few basic types of reactions that involve the transfer of functional groups (Mitchell 1979). This common chemistry allows cells to use a small set of metabolic intermediates to carry chemical groups between different reactions (Wimmer and Rose 1978). These group-transfer intermediates are the coenzymes.
Each class of group-transfer reaction is carried out by a particular coenzyme, which is the substrate for a set of enzymes that produce it, and a set of enzymes that consume it. An example of this are the dehydrogenases that use nicotinamide adenine dinucleotide (NADH) as a cofactor. Here, hundreds of separate types of enzymes remove electrons from their substrates and reduce NAD+ to NADH. This reduced coenzyme is then a substrate for any of the reductases in the cell that need to reduce their substrates (Pollak et al. 2007).
Coenzymes are therefore continuously recycled as part of metabolism. As an example, the total quantity of ATP in the human body is about 0.1 mole. This ATP is constantly being broken down into ADP, and then converted back into ATP. Thus, at any given time, the total amount of ATP + ADP remains fairly constant. The energy used by human cells requires the hydrolysis of 100 to 150 moles of ATP daily, which is around 50 to 75 kilograms. Typically, a human will use up their body weight of ATP over the course of the day (Di Carlo and Collins 2001). This means that each ATP molecule is recycled 1000 to 1500 times daily.
Types
Acting as coenzymes in organisms is the major role of vitamins, although vitamins do have other functions in the body (Bolander 2006). Coenzymes are also commonly made from nucleotides, such as adenosine triphosphate, the biochemical carrier of phosphate groups, or coenzyme A, the coenzyme that carries acyl groups. Most coenzymes are found in a huge variety of species, and some are universal to all forms of life. An exception to this wide distribution is a group of unique coenzymes that evolved in methanogens, which are restricted to this group of archaea (Rouvière and Wolfe 1988).
Vitamins and derivatives
Coenzyme | Vitamin | Additional component | Chemical group(s) transferred | Distribution |
NAD+ and NADP+ [1] | Niacin (B3) | ADP | Electrons | Bacteria, archaea, and eukaryotes |
Coenzyme A [2] | Pantothenic acid (B5) | ADP | Acetyl group and other acyl groups | Bacteria, archaea and eukaryotes |
Tetrahydrofolic acid [3] | Folic acid (B9) | Glutamate residues | Methyl, formyl, methylene and formimino groups | Bacteria, archaea and eukaryotes |
Menaquinone [4] | Vitamin K | None | Carbonyl group and electrons | Bacteria, archaea and eukaryotes |
Ascorbic acid [5] | Vitamin C | None | Electrons | Bacteria, archaea and eukaryotes |
Coenzyme F420 [6] | Riboflavin (B2) | Amino acids | Electrons | Methanogens and some bacteria |
Non-vitamins
Coenzyme | Chemical group(s) transferred | Distribution |
Adenosine triphosphate [7] | Phosphate group | Bacteria, archaea and eukaryotes |
S-Adenosyl methionine [8] | Methyl group | Bacteria, archaea and eukaryotes |
3'-Phosphoadenosine-5'-phosphosulfate [9] | Sulfate group | Bacteria, archaea and eukaryotes |
Coenzyme Q [10] | Electrons | Bacteria, archaea, and eukaryotes |
Tetrahydrobiopterin [11] | Oxygen atom and electrons | Bacteria, archaea, and eukaryotes |
Cytidine triphosphate [12] | Diacylglycerols and lipid head groups | Bacteria, archaea, and eukaryotes |
Nucleotide sugars [13] | Monosaccharides | Bacteria, archaea, and eukaryotes |
Glutathione [14] | Electrons | Some bacteria and most eukaryotes |
Coenzyme M [15] | Methyl group | Methanogens |
Coenzyme B [16] | Electrons | Methanogens |
Methanofuran [17] | Formyl group | Methanogens |
Tetrahydromethanopterin [18] | Methyl group | Methanogens |
History
The first coenzyme to be discovered was NAD+, which was identified by Arthur Harden and William Youndin and reported on in 1906 (Harden and Young 1906). They noticed that adding boiled and filtered yeast extract greatly accelerated alcoholic fermentation in unboiled yeast extracts. They called the unidentified factor responsible for this effect a coferment. Through a long and difficult purification from yeast extracts, this heat-stable factor was identified as a nucleotide sugar phosphate by Hans von Euler-Chelpin (1930). Other coenzymes were identified throughout the early twentieth century, with ATP being isolated in 1929, by Karl Lohmann (1929), and coenzyme A being discovered in 1945, by Fritz Albert Lipmann (1945).
The functions of coenzymes were at first mysterious, but in 1936, Otto Heinrich Warburg identified the function of NAD+ in hydride transfer (Warburg and Christian (1936). This discovery was followed in the early 1940s by the work of Herman Kalckar, who established the link between the oxidation of sugars and the generation of ATP (Kalckar 1974). This confirmed the central role of ATP in energy transfer that had been proposed by Fritz Albert Lipmann in 1941 (Lipmann (1941). Later, in 1949, Morris Friedkin and Albert L. Lehninger proved that the coenzyme NAD+ linked metabolic pathways, such as the citric acid cycle and the synthesis of ATP (Friedkin and Lehninger 1949).
Evolution
Coenzymes, such as ATP and NADH, are present in all known forms of life and form a core part of metabolism. Such universal conservation indicates that these molecules evolved very early in the development of living things (Chen et al. 2007). At least some of the current set of coenzymes may therefore have been present in the last universal ancestor, which lived about 4 billion years ago (Koch 1998; Ouzounis and Kyrpides 1996).
Coenzymes may have been present even earlier in the history of life on Earth (White 1976). Interestingly, the nucleotide adenosine is present in coenzymes that catalyse many basic metabolic reactions such as methyl, acyl, and phosphoryl group transfer, as well as redox reactions. This ubiquitous chemical scaffold has therefore been proposed to be a remnant of the RNA world, with early ribozymes evolving to bind a restricted set of nucleotides and related compounds (Saran et al. 2003; Jadhav and Yarus 2002). Adenosine-based coenzymes are thought to have acted as interchangeable adaptors that allowed enzymes and ribozymes to bind new coenzymes through small modifications in existing adenosine-binding domains, which had originally evolved to bind a different cofactor (Denessiouk et al. 2001). This process of adapting a pre-evolved structure for a novel use is referred to as exaptation.
Notes
- ↑ Pollak et al. (2007)
- ↑ Leonardi et al. (2005).
- ↑ Donnelly (2001)
- ↑ Søballe and Poole (1999).
- ↑ Linster and Van Schaftingen (2007)
- ↑ Mack and Grill (2006).
- ↑ Knowles (1980).
- ↑ Chiang et al. (1996).
- ↑ Negishi et al. (2001).
- ↑ Crane (2001)
- ↑ Thony et al. (2000).
- ↑ Buchanan et al. (2000)
- ↑ Ginsburg (1978).
- ↑ Grill et al. (2001); Meister and Anderson (1983).
- ↑ Taylor and Wolfe (1974); Balch and Wolfe (1979).
- ↑ Noll et al. 1986).
- ↑ Vorholt and Thauer (1997).
- ↑ DiMarco et al. (1990).
ReferencesISBN links support NWE through referral fees
- Alberts, B., D. Bray, J. Lewis, M. Raff, K. Roberts, and J.D. Watson. Molecular Biology of the Cell, 2nd edition. New York: Garland Publishing, 1989. ISBN 0824036956.
- Balch, W.E., and R.S. Wolfe. 1979. Specificity and biological distribution of coenzyme M (2-mercaptoethanesulfonic acid). J. Bacteriol. 137(1): 256–63. PMID 104960. Retrieved August 12, 2008.
- Bender, D.A., and A.E. Bender. 2005. A Dictionary of Food and Nutrition. New York: Oxford University Press. ISBN 0198609612.
- Biocarta Leloir pathway of galactose metabolism. Charting pathways of life. Retrieved August 4, 2008.
- Bolander, F.F. 2006. Vitamins: Not just for enzymes. Curr Opin Investig Drugs 7(10): 912–5. PMID 17086936.
- Buchanan, B.B., W. Gruissem, and R.L. Jones. 2000. Biochemistry & Molecular Biology of Plants, 1st edition. American Society of Plant Physiology. ISBN 0943088399.
- Chen, X., N. Li, and A. D. Ellington. 2007. Ribozyme catalysis of metabolism in the RNA world. Chem. Biodivers. 4(4): 633–55. PMID 17443876. Retrieved August 12, 2008.
- Chiang, P., R. Gordon, J. Tal, G. Zeng, B. Doctor, and K. Pardhasaradhi, and P. McCann. 1996. S-Adenosylmethionine and methylation. FASEB J 10(4): 471–80. PMID 8647346. Retrieved August 12, 2008.
- Crane, F.L. 2001. Biochemical functions of coenzyme Q10. Journal of the American College of Nutrition 20(6): 591-8. PMID 11771674. Retrieved August 12, 2008.
- Denessiouk, K.A., V.V. Rantanen, and M.S. Johnson. 2001. Adenine recognition: A motif present in ATP-, CoA-, NAD-, NADP-, and FAD-dependent proteins. Proteins 44(3): 282–91. PMID 11455601. Retrieved August 12, 2008.
- Di Carlo, S.E., and H.L. Collins. 2001. Estimating ATP resynthesis during a marathon run: A method to introduce metabolism. Advan. Physiol. Edu. 25: 70-71. Retrieved August 12, 2008.
- DiMarco, A. A., T. A. Bobik, and R. S. Wolfe. 1990. Unusual coenzymes of methanogenesis. Annu. Rev. Biochem. 59: 355–94. PMID 2115763. Retrieved August 12, 2008.
- Donnelly, J. G. 2001. Folic acid. Crit Rev Clin Lab Sci 38(3): 183–223. PMID 11451208. Retrieved August 12, 2008.
- Friedkin, M., and A.L. Lehninger. 1949. Esterification of inorganic phosphate coupled to electron transport between dihydrodiphosphopyridine nucleotide and oxygen. J. Biol. Chem. 178(2): 611–23. PMID 18116985. Retrieved August 12, 2008.
- Ginsburg, V. 1978. Comparative biochemistry of nucleotide-linked sugars. Prog. Clin. Biol. Res. 23: 595–600. PMID 351635.
- Grill, D., T. Tausz, and L.J. De Kok. 2001. Significance of glutathione in plant adaptation to the environment. Springer. ISBN 1402001789. Retrieved August 12,2008.
- Harden, A., and W.J. Young. 1906. The alcoholic ferment of yeast-juice. Proceedings of the Royal Society of London. Series B, Containing Papers of a Biological Character 78(526): 369-375.
- International Union of Pure and Applied Chemistry (IUPAC), Inorganic Chemistry Division, Commission on Nomenclature of Inorganic Chemistry. 1997a. Glossary of terms used in bioinorganic chemistry: Coenzyme. From M. W. G. de Bolster, Pure Appl. Chem. 69: 1251-1303. Retrieved August 12, 2008.
- —. 1997b. Glossary of terms used in bioinorganic chemistry: Prosthetic group. From M. W. G. de Bolster, Pure Appl. Chem. 69: 1251-1303. Retrieved August 12, 2008.
- —. 1997c. Glossary of terms used in bioinorganic chemistry: Cofactor. From M. W. G. de Bolster, Pure Appl. Chem. 69: 1251-1303. Retrieved August 12, 2008.
- Jadhav, V. R., and M. Yarus. 2002. Coenzymes as coribozymes. Biochimie 84(9): 877–88. PMID 12458080.
- Kalckar, H. M. 1974. Origins of the concept oxidative phosphorylation. Mol. Cell. Biochem. 5(1–2): 55-63. PMID 4279328. Retrieved August 12, 2008.
- Knowles, J. R. 1980. Enzyme-catalyzed phosphoryl transfer reactions. Annu. Rev. Biochem. 49: 877–919. PMID 6250450. Retrieved August 12, 2008.
- Koch, A. 1998. How did bacteria come to be? Adv Microb Physiol 40: 353–99. PMID 9889982. Retrieved August 12, 2008.
- Leonardi, R., Y. M. Zhang, C. O. Rock, and S. Jackowski. 2005. Coenzyme A: Back in action. Prog. Lipid Res. 44(2-3): 125–53. PMID 15893380. Retrieved August 12, 2008.
- Linster, C. L., and E. Van Schaftingen. 2007. Vitamin C. Biosynthesis, recycling and degradation in mammals. FEBS J. 274(1): 1–22. PMID 17222174. Retrieved August 12, 2008.
- Lipmann, F. 1945. Acetylation of sulfanilamide by liver homogenates and extracts. J. Biol. Chem. 160(1): 173–190. Retrieved August 12, 2008.
- Lipmann, F. 1941. Metabolic generation and utilization of phosphate bond energy. Adv Enzymol 1: 99-162.
- Lohmann, K. 1929. Über die Pyrophosphatfraktion im Muskel. Naturwissenschaften 17: 624–625.
- Mack, M., and S. Grill. 2006. Riboflavin analogs and inhibitors of riboflavin biosynthesis. Appl. Microbiol. Biotechnol. 71(3): 265–75. PMID 16607521. Retrieved August 12, 2008.
- McGraw-Hill Concise Encyclopedia of Science & Technology. 2005. New York: McGraw-Hill. ISBN 0071429573.
- Meister, A., and M. E. Anderson. 1983. Glutathione. Annu. Rev. Biochem. 52: 711–60. PMID 6137189. Retrieved August 12, 2008.
- Mitchell, P. 1979. The Ninth Sir Hans Krebs Lecture. Compartmentation and communication in living systems. Ligand conduction: a general catalytic principle in chemical, osmotic and chemiosmotic reaction systems. Eur J Biochem 95(1): 1-20. PMID 378655. Retrieved August 12, 2008.
- Negishi, M., L.G. Pedersen, E. Petrotchenko, et al. 2001. Structure and function of sulfotransferases. Arch. Biochem. Biophys. 390(2): 149–57. PMID 11396917. Retrieved August 12, 2008.
- Noll, K.M., K.L. Rinehart, R.S. Tanner, and R.S. Wolfe. 1986. Structure of component B (7-mercaptoheptanoylthreonine phosphate) of the methylcoenzyme M methylreductase system of Methanobacterium thermoautotrophicum. Proc. Natl. Acad. Sci. U.S.A. 83(12): 4238–42. PMID 3086878. Retrieved August 12, 2008.
- Ouzounis, C., and N. Kyrpides. 1996. The emergence of major cellular processes in evolution. FEBS Lett 390(2): 119-23. PMID 8706840. Retrieved August 12, 2008.
- Pollak, N., C. Dölle, and M. Ziegler. 2007. The power to reduce: Pyridine nucleotides—small molecules with a multitude of functions. Biochem. J. 402(2): 205-18. PMID 17295611. Retrieved August 12, 2008.
- Rouvière, P.E., and R.S. Wolfe. 1988. Novel biochemistry of methanogenesis. J. Biol. Chem. 263(17): 7913–6. PMID 3131330. Retrieved August 12, 2008.
- Saran, D., J. Frank, and D. H. Burke. 2003. The tyranny of adenosine recognition among RNA aptamers to coenzyme A. BMC Evol. Biol. 3: 26. PMID 14687414. Retrieved August 12, 2008.
- Søballe, B., and R. K. Poole. 1999. Microbial ubiquinones: Multiple roles in respiration, gene regulation and oxidative stress management. Microbiology 145: 1817-30. PMID 10463148. Retrieved August 12, 2008.
- Taylor, C. D., and R. S. Wolfe. 1974. Structure and methylation of coenzyme M(HSCH2CH2SO3). J. Biol. Chem. 249(15): 4879–85. PMID 4367810. Retrieved August 12, 2008.
- Thony, B., G. Auerbach, and N. Blau. 2000. Tetrahydrobiopterin biosynthesis, regeneration and functions. Biochem J 347: 1–16. PMID 10727395. Retrieved August 12, 2008.
- von Euler-Chelpin, H. 1930. Fermentation of sugars and fermentative enzymes: Nobel Lecture, May 23, 1930. Nobel Foundation. Retrieved August 12, 2008.
- Vorholt, J. A., and R. K. Thauer. 1997. The active species of 'CO2' utilized by formylmethanofuran dehydrogenase from methanogenic Archaea. Eur. J. Biochem. 248(3): 919–24. PMID 9342247. Retrieved August 12, 2008.
- Warburg, O., and W. Christian. 1936. Pyridin, the hydrogen-transferring component of the fermentation enzymes (pyridine nucleotide). Biochemische Zeitschrift 287: 291.
- White, H. B. 1976. Coenzymes as fossils of an earlier metabolic state. J. Mol. Evol. 7(2): 101–4. PMID 1263263. Retrieved August 12, 2008.
- Wimmer, M., and I. Rose. 1978. Mechanisms of enzyme-catalyzed group transfer reactions. Annu Rev Biochem 47: 1031-78. PMID 354490. Retrieved August 12, 2008.
|
Credits
New World Encyclopedia writers and editors rewrote and completed the Wikipedia article in accordance with New World Encyclopedia standards. This article abides by terms of the Creative Commons CC-by-sa 3.0 License (CC-by-sa), which may be used and disseminated with proper attribution. Credit is due under the terms of this license that can reference both the New World Encyclopedia contributors and the selfless volunteer contributors of the Wikimedia Foundation. To cite this article click here for a list of acceptable citing formats.The history of earlier contributions by wikipedians is accessible to researchers here:
The history of this article since it was imported to New World Encyclopedia:
Note: Some restrictions may apply to use of individual images which are separately licensed.