Difference between revisions of "Glycogen" - New World Encyclopedia
(26 intermediate revisions by 8 users not shown) | |||
Line 1: | Line 1: | ||
− | + | {{2Copyedited}}{{Ebcompleted}}{{Copyedited}}{{Paid}}{{Approved}}{{Images OK}}{{Submitted}} | |
− | + | [[Image:Glycogen.png|thumb|right|300px|The structure of glycogen. Most glucose residues are linked by α-1,4 glycosidic bonds (labeled at top). Approximately one in ten glucose residues form α-1,6 glycosidic bonds, creating a branched structure (green). The non-reducing end-branches (red) facilitate glycogen's interaction with enzymes involved in its synthesis and breakdown.]] | |
− | Glycogen is | + | '''Glycogen''' is the principal storage form of [[glucose]] (Glc) in [[animal]] [[cell (biology)|cells]], though it is also found in various [[species]] of [[microorganism]]s, such as [[bacteria]] and [[fungi]]. It is a large, branched polymer of linked glucose residues (portions of larger molecules) that can be readily mobilized as an energy source, increasing the amount of glucose immediately available to the organism (1) between meals and (2) during muscular activity. Since the [[brain]] relies on glucose as its preferred fuel, the ability to maintain a steady supply of glucose, which is the major [[sugar]] circulating in the [[blood]] of higher animals, is crucial to survival. |
− | Glycogen is | + | Glycogen is found in the form of granules in the cytosol, the internal fluid of the cell. About three-fourths of the body’s glycogen supply is stored in [[muscle]] cells. However, [[liver]] cells (hepatocytes) have the highest concentration of glucose (a maximum of approximately eight percent in liver versus one percent of the muscle mass of an adult male [[human being]]). Small amounts of glycogen are also found in the [[kidney]]s, and even smaller amounts in certain glial cells in the brain and in white blood cells. |
− | |||
− | |||
− | [[Glycogen-storage disorders | + | The [[physiology|physiological]] role of glycogen depends on the type of cell in which it is stored: |
+ | *[[Liver]] cells play a key role in regulating the blood glucose level as they can either break down glycogen (glycogenolysis) to release glucose into the blood or withdraw glucose from the blood and store it by synthesizing glycogen (glycogenesis). It is noteworthy that glucose is not a major fuel for the liver, which mainly utilizes keto acids. The liver cells, therefore, perform the glucose storage and release primarily for the benefit of other organs. This reflects the principle of [[Biology#Interactions: Harmony and dual purposes|dual purposes]], whereby the components of living organisms work together harmoniously because they not only exhibit an individual purpose oriented toward their own self-maintenance and development, but also serve a purpose for the whole. | ||
+ | |||
+ | *In skeletal [[muscle]], glycogen is an energy reserve that can be tapped during exercise. Muscle cells lack the ability to release glucose into the blood, so their glycogen store is destined for internal use, powering muscle contraction during strenuous activity. | ||
+ | |||
+ | Glycogen-storage disorders are a type of inherited [[metabolic disease]] resulting from deficiencies of the [[enzyme]]s that participate in glycogen metabolism. Symptoms vary in type and severity, ranging from exercise intolerance to low blood sugar and kidney disease. Certain forms of glycogen-storage disorders cause cardio-respiratory failure or liver failure in affected infants. | ||
==Glycogen's branched structure makes it an accessible energy source== | ==Glycogen's branched structure makes it an accessible energy source== | ||
− | |||
− | Glycogen is a highly | + | Glycogen is a highly branched [[polymer]] of about 30,000 glucose residues. It has a molecular weight between 10<sup>6</sup> and 10<sup>7</sup> daltons. Given its size, glycogen is considered a [[Carbohydrate#Polysaccharides|polysaccharide]]: i.e., a large [[carbohydrate]] constructed out of hundreds or thousands of linked [[Carbohydrate#monosaccharides|monosaccharides]] (such as glucose). |
− | + | Linking the monosaccharide components of glycogen are glycosidic bonds, chemical bonds that form between the hemiacetal group of a saccharide and the hydroxyl group of an [[alcohol]]. Specifically, most of the glucose units are linked by α-1,4 bonds, in which the carbon-1 of one sugar molecule is linked to the carbon-4 of the adjacent molecule. In the alpha configuration, the oxygen atom is located below the plane of the sugar ring. | |
− | The branches increase the solubility of glycogen and make its sugar units accessible | + | Approximately one in ten glucose residues also forms an α-1,6 glycosidic bond with an adjacent glucose, which results in the creation of a branch. Glycogen has only one reducing end and a large number of non-reducing ends with a free hydroxyl group at carbon-4. The branches increase the solubility of glycogen and make its sugar units accessible to the [[enzyme]]s involved in glycogen [[metabolism]], which nest between the outer branches of the glycogen molecules and act on the non-reducing ends. Therefore, the many end-branches of glycogen facilitate its rapid synthesis and breakdown, making it a readily mobilized source of energy. |
− | [[Starch]], which plays a similar energy-storage role in | + | [[Starch]], which plays a similar energy-storage role in [[plant]]s, can also exist in a branched form called amylopectin, though it has a lesser degree of branching than glycogen (about one in 30 glucose residues form α-1,6 bonds). In contrast, [[cellulose]], the other major polysaccharide in plants, is an unbranched polymer of glucose, in which β-1,4 linkages form very long, straight chains. This closed structure is suited to the structural role of cellulose, a major component of plant cell walls, whereas the open helices of glycogen and starch, which are nutritional molecules, provide easy access to stored glucose. |
==Glycogen in liver functions to maintain blood sugar levels== | ==Glycogen in liver functions to maintain blood sugar levels== | ||
− | The liver is a major control site of blood glucose levels; it | + | The [[liver]] is a major control site of blood glucose levels; it responds to [[hormone|hormonal]] signals that indicate reduced or elevated amounts of glucose in the blood. The synthesis and breakdown of glycogen in the liver thus serves as a means for maintaining a steady supply of fuel for organs such as the [[brain]], allowing glucose to be stored or released depending on the energy needs of the organism. |
− | As a [[carbohydrate]] meal is eaten and digested, blood glucose levels rise, and the [[pancreas]] secretes the hormone [[insulin]]. | + | As a [[carbohydrate]] meal is eaten and digested, blood glucose levels rise, and the [[pancreas]] secretes the hormone [[insulin]]. The hepatic portal vein delivers glucose-rich blood from the digestive system to the liver’s hepatocytes; insulin, also carried in the blood, acts on the hepatocytes to stimulate the action of several [[enzyme]]s, including glycogen synthase, involved in the synthesis of glycogen. Glucose molecules are added to the chains of glycogen for as long as both insulin and glucose remain plentiful. In this postprandial or "fed" state, the liver takes in more glucose from the blood than it releases. |
− | [[ | + | The hormones [[glucagon]], produced by the pancreas, and [[epinephrine]], secreted by the [[adrenal gland]], serve in many respects as a counter-signal to insulin. When blood glucose levels begin to fall (about four hours after a meal), they stimulate the breakdown of glycogen. The freed glucose is then released from the liver into the blood. For the next eight to 12 hours (for example, during an overnight fast), glucose derived from liver glycogen will be the primary source of blood glucose to be used by the rest of the body for fuel. |
− | + | Although liver cells maintain a high concentration of glycogen, the liver meets most of its own energy needs through keto acids derived from the breakdown of [[amino acid]]s. The liver's role in glycogen metabolism is to synthesize and degrade glycogen for the benefit of the organism as a whole. | |
==Glycogen in muscle is an energy reserve for strenuous exercise== | ==Glycogen in muscle is an energy reserve for strenuous exercise== | ||
− | + | [[Muscle]] cells lack the [[enzyme]] ''glucose-6-phosphatase'', which is the enzyme that enables liver cells to export glucose into the blood. Therefore, the glycogen stored in muscle cells is utilized internally rather than shared. Other cells that contain small amounts of glycogen use it locally as well. | |
− | + | Glycogen in muscle cells functions as an immediate source of available glucose during bursts of activity, such as a 100-meter sprint. When the energy needs of the cell outpace its limited oxygen supply, [[ATP]] (the "energy currency" of the cell) is produced in part by the anaerobic [[glycolysis]] of glucose derived from muscle glycogen. Glycolysis is a metabolic pathway by which glucose may be broken down to [[pyruvate]] in the absence of oxygen. Although the complete oxidation of glucose in the presence of oxygen ([[oxidative phosphorylation]]) produces about 18 times the amount of ATP, glycolysis occurs at a rate approximately 100 times faster than aerobic respiration. During a period of brief, intense exertion, the energy requirement is to generate the maximum amount of ATP for muscle contraction in the shortest time frame. However, a longer period of activity requires at least the partial use of ATP derived from oxidative phosphorylation, which explains the slower pace of a 1,000-meter run. | |
− | [[ | + | [[Image:Cori cycle.PNG|right|thumb|360px|A schematic depicting the Cori cycle]] |
+ | The liver may also work in tandem with skeletal muscle in times of exertion. The '''Cori cycle''' refers to the recycling of [[lactate]] or lactic acid produced by muscle during anaerobic metabolism. The lactate is converted to glucose by the liver. This permits the regeneration of NAD<sup>+</sup> required for glycolysis to continue. The lactate diffuses into the blood and is taken up by the liver, which oxidizes it back to [[pyruvate]]. Most of the pyruvate is then converted to glucose (via gluconeogenesis). This glucose circulates in the blood, where it can be used by muscles if needed or stored as glycogen. The Cori cycle allows the muscles to continue focusing exclusively on the production of ATP while the liver handles the lactate produced in muscle. The cycle also prevents lactate acidosis by removing lactate from the blood. Otherwise, [[pH]] would fall as the buffering capacity of blood is exceeded. | ||
===Glycogen and marathon running=== | ===Glycogen and marathon running=== | ||
− | + | Since the [[human body]] is unable to hold more than approximately 2,000 kcal of glycogen, marathon runners commonly experience a phenomenon referred to as "bonking" or "hitting the wall" around the 20-mile (32-km) point of a marathon. Symptoms of this condition, which signals the depletion of glycogen stores, include general weakness, fatigue, and manifestations of hypoglycemia (low blood sugar), such as dizziness and even hallucinations. This rapid drop in performance results from a shift in the fuel supply: as glycogen stores diminish, [[ATP]] must also be generated in part from [[fatty acid]] oxidation, which is a slower process than the oxidation of glycogen. The simultaneous utilization of both fuels allows for a balance between endurance and speed, preserving enough glucose to fuel the runner's final push to the finish line. | |
− | + | There are several approaches available to prevent glycogen depletion during a marathon or another endurance exercise such as cycling: | |
+ | |||
+ | *Carbohydrate loading is used to ensure that the initial glycogen level is maximized. This technique consists of increasing the intake of complex carbohydrates in the final three days preceding the event. | ||
+ | *Consuming food or drink that contains carbohydrates during the exercise will replenish the supply of glucose. This is a requirement for very long distances; it is estimated that Tour de France competitors receive up to 50 percent of their daily caloric intake from on-the-bike supplements. | ||
+ | *Decreasing the intensity of the exercise to the so-called "fat-loss" level (a heart rate of 130 beats per minute for a 30-year-old athlete) will lower both the energy requirements per unit of distance and the fraction of the energy that comes from glycogen. | ||
==Disorders of glycogen metabolism== | ==Disorders of glycogen metabolism== | ||
− | The most common disease | + | The most common [[disease]] involving abnormal glycogen metabolism is [[diabetes mellitus]], which is characterized by persistent variable [[hyperglycemia]] (high blood sugar levels), resulting either from a deficiency of [[insulin]] or from an inadequate response by the body's cells to insulin. As mentioned above, insulin is the principal control signal for the conversion of glucose to glycogen for storage in [[liver]] and [[muscle]] cells. Lowered insulin levels result in the reverse conversion of glycogen to glucose by the liver when blood sugar levels fall. With the system out of balance, the liver then releases more glucose into the blood than can be utilized by other cells. |
− | + | Several inborn errors of metabolism are caused by inherited genetic deficiencies of the enzymes involved in glycogen synthesis or breakdown. Collectively referred to as [[Metabolic disease|glycogen storage disease]]s, they include the following types: | |
− | + | *'''von Gierke's disease''' (Type I) is the most common of the glycogen storage diseases. It results from a deficiency of the enzyme glucose-6-phosphatase, which in turn impairs the ability of the liver to produce free glucose from glycogen stores and through gluconeogenesis. Since these are the two primary [[metabolism|metabolic]] mechanisms by which the liver supplies glucose to the rest of the body during periods of [[fasting]], hypoglycemia is symptomatic of the disease. Reduced glycogen breakdown results in increased glycogen storage in liver and kidneys, causing enlargement of both organs. Frequent or continuous feedings of cornstarch or other [[carbohydrate]]s is the principal treatment. | |
+ | *'''Pompe disease''' (Type II) is caused by a deficiency in a [[lysosome]]-specific enzyme of glycogen breakdown called ''acid alpha-glucosidase'' (GAA). It is the only glycogen storage disease involving a defect in the lysosome, an organelle that contains digestive enzymes that breakdown macromolecules such as glycogen. The resulting build-up of glycogen causes progressive muscle weakness (myopathy) throughout the body and affects various body tissues, particularly in the heart, skeletal muscles, liver, and nervous system. | ||
+ | *A disorder involving glycogen metabolism in muscle is '''McArdle's disease''' (Type V). It is characterized by a deficiency of ''myophosphorylase'', the muscle isoform of the enzyme ''glycogen phosphorylase''. This enzyme participates in the breakdown of glycogen so that it can be utilized within the muscle cell. Persons with this disease experience difficulty when their muscles are called upon to perform relatively brief yet intense activity. The inability to break down glycogen into glucose leads to an energy shortage within the muscle, resulting in muscle pain and cramping, and sometimes causing serious injury to the muscles. In addition, the breakdown of muscle tissue can indirectly lead to [[kidney]] damage. Anaerobic exercise must be avoided but gentle aerobic activity is beneficial. | ||
==References== | ==References== | ||
− | Stryer, | + | * Chen Y.-T., and A. Burchell. “Glycogen storage diseases.” In C. R. Scriver, A. L. Beaudet, W. S. Sly, and D. Valle (eds.), ''The Metabolic Basis of Inherited Disease'', 7th edition. New York: McGraw-Hill, 1995. |
− | + | * Cornblath M, and R. Schwartz. “Disorders of glycogen metabolism.” In M. Cornblath and R. Schwartz, ''Disorders of Carbohydrate Metabolism in Infancy'', 3rd edition. Cambridge: Blackwell, 1991. | |
− | + | * Stryer, L. 1995. ''Biochemistry'', 4th edition. New York: W. H. Freeman. | |
− | * | + | * Tsalikian E., and M. W. Haymond. “Hypoglycemia in infants and children.” In F. J. Service, ''Hypoglycemic Disorders: Pathogenesis, Diagnosis, and Treatment''. Boston: G. K. Hall Medical Publishers, 1983. |
− | |||
− | |||
− | {{ | + | {{credit7|Glycogen|64487380|Cori_cycle|73398486|Glycogen_storage_disease_type_I|70668855|Glycogen_storage_disease_type_II|72171805|Glycogen_storage_disease_type_V|65114935|Bonk_(condition)|67982729|Glycosidic_bond|71667268}} |
[[Category:Life sciences]] | [[Category:Life sciences]] | ||
+ | [[Category:Biochemistry]] |
Latest revision as of 18:24, 30 August 2021
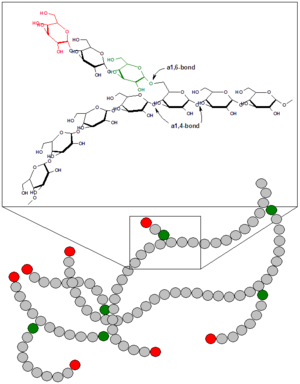
Glycogen is the principal storage form of glucose (Glc) in animal cells, though it is also found in various species of microorganisms, such as bacteria and fungi. It is a large, branched polymer of linked glucose residues (portions of larger molecules) that can be readily mobilized as an energy source, increasing the amount of glucose immediately available to the organism (1) between meals and (2) during muscular activity. Since the brain relies on glucose as its preferred fuel, the ability to maintain a steady supply of glucose, which is the major sugar circulating in the blood of higher animals, is crucial to survival.
Glycogen is found in the form of granules in the cytosol, the internal fluid of the cell. About three-fourths of the body’s glycogen supply is stored in muscle cells. However, liver cells (hepatocytes) have the highest concentration of glucose (a maximum of approximately eight percent in liver versus one percent of the muscle mass of an adult male human being). Small amounts of glycogen are also found in the kidneys, and even smaller amounts in certain glial cells in the brain and in white blood cells.
The physiological role of glycogen depends on the type of cell in which it is stored:
- Liver cells play a key role in regulating the blood glucose level as they can either break down glycogen (glycogenolysis) to release glucose into the blood or withdraw glucose from the blood and store it by synthesizing glycogen (glycogenesis). It is noteworthy that glucose is not a major fuel for the liver, which mainly utilizes keto acids. The liver cells, therefore, perform the glucose storage and release primarily for the benefit of other organs. This reflects the principle of dual purposes, whereby the components of living organisms work together harmoniously because they not only exhibit an individual purpose oriented toward their own self-maintenance and development, but also serve a purpose for the whole.
- In skeletal muscle, glycogen is an energy reserve that can be tapped during exercise. Muscle cells lack the ability to release glucose into the blood, so their glycogen store is destined for internal use, powering muscle contraction during strenuous activity.
Glycogen-storage disorders are a type of inherited metabolic disease resulting from deficiencies of the enzymes that participate in glycogen metabolism. Symptoms vary in type and severity, ranging from exercise intolerance to low blood sugar and kidney disease. Certain forms of glycogen-storage disorders cause cardio-respiratory failure or liver failure in affected infants.
Glycogen's branched structure makes it an accessible energy source
Glycogen is a highly branched polymer of about 30,000 glucose residues. It has a molecular weight between 106 and 107 daltons. Given its size, glycogen is considered a polysaccharide: i.e., a large carbohydrate constructed out of hundreds or thousands of linked monosaccharides (such as glucose).
Linking the monosaccharide components of glycogen are glycosidic bonds, chemical bonds that form between the hemiacetal group of a saccharide and the hydroxyl group of an alcohol. Specifically, most of the glucose units are linked by α-1,4 bonds, in which the carbon-1 of one sugar molecule is linked to the carbon-4 of the adjacent molecule. In the alpha configuration, the oxygen atom is located below the plane of the sugar ring.
Approximately one in ten glucose residues also forms an α-1,6 glycosidic bond with an adjacent glucose, which results in the creation of a branch. Glycogen has only one reducing end and a large number of non-reducing ends with a free hydroxyl group at carbon-4. The branches increase the solubility of glycogen and make its sugar units accessible to the enzymes involved in glycogen metabolism, which nest between the outer branches of the glycogen molecules and act on the non-reducing ends. Therefore, the many end-branches of glycogen facilitate its rapid synthesis and breakdown, making it a readily mobilized source of energy.
Starch, which plays a similar energy-storage role in plants, can also exist in a branched form called amylopectin, though it has a lesser degree of branching than glycogen (about one in 30 glucose residues form α-1,6 bonds). In contrast, cellulose, the other major polysaccharide in plants, is an unbranched polymer of glucose, in which β-1,4 linkages form very long, straight chains. This closed structure is suited to the structural role of cellulose, a major component of plant cell walls, whereas the open helices of glycogen and starch, which are nutritional molecules, provide easy access to stored glucose.
Glycogen in liver functions to maintain blood sugar levels
The liver is a major control site of blood glucose levels; it responds to hormonal signals that indicate reduced or elevated amounts of glucose in the blood. The synthesis and breakdown of glycogen in the liver thus serves as a means for maintaining a steady supply of fuel for organs such as the brain, allowing glucose to be stored or released depending on the energy needs of the organism.
As a carbohydrate meal is eaten and digested, blood glucose levels rise, and the pancreas secretes the hormone insulin. The hepatic portal vein delivers glucose-rich blood from the digestive system to the liver’s hepatocytes; insulin, also carried in the blood, acts on the hepatocytes to stimulate the action of several enzymes, including glycogen synthase, involved in the synthesis of glycogen. Glucose molecules are added to the chains of glycogen for as long as both insulin and glucose remain plentiful. In this postprandial or "fed" state, the liver takes in more glucose from the blood than it releases.
The hormones glucagon, produced by the pancreas, and epinephrine, secreted by the adrenal gland, serve in many respects as a counter-signal to insulin. When blood glucose levels begin to fall (about four hours after a meal), they stimulate the breakdown of glycogen. The freed glucose is then released from the liver into the blood. For the next eight to 12 hours (for example, during an overnight fast), glucose derived from liver glycogen will be the primary source of blood glucose to be used by the rest of the body for fuel.
Although liver cells maintain a high concentration of glycogen, the liver meets most of its own energy needs through keto acids derived from the breakdown of amino acids. The liver's role in glycogen metabolism is to synthesize and degrade glycogen for the benefit of the organism as a whole.
Glycogen in muscle is an energy reserve for strenuous exercise
Muscle cells lack the enzyme glucose-6-phosphatase, which is the enzyme that enables liver cells to export glucose into the blood. Therefore, the glycogen stored in muscle cells is utilized internally rather than shared. Other cells that contain small amounts of glycogen use it locally as well.
Glycogen in muscle cells functions as an immediate source of available glucose during bursts of activity, such as a 100-meter sprint. When the energy needs of the cell outpace its limited oxygen supply, ATP (the "energy currency" of the cell) is produced in part by the anaerobic glycolysis of glucose derived from muscle glycogen. Glycolysis is a metabolic pathway by which glucose may be broken down to pyruvate in the absence of oxygen. Although the complete oxidation of glucose in the presence of oxygen (oxidative phosphorylation) produces about 18 times the amount of ATP, glycolysis occurs at a rate approximately 100 times faster than aerobic respiration. During a period of brief, intense exertion, the energy requirement is to generate the maximum amount of ATP for muscle contraction in the shortest time frame. However, a longer period of activity requires at least the partial use of ATP derived from oxidative phosphorylation, which explains the slower pace of a 1,000-meter run.
The liver may also work in tandem with skeletal muscle in times of exertion. The Cori cycle refers to the recycling of lactate or lactic acid produced by muscle during anaerobic metabolism. The lactate is converted to glucose by the liver. This permits the regeneration of NAD+ required for glycolysis to continue. The lactate diffuses into the blood and is taken up by the liver, which oxidizes it back to pyruvate. Most of the pyruvate is then converted to glucose (via gluconeogenesis). This glucose circulates in the blood, where it can be used by muscles if needed or stored as glycogen. The Cori cycle allows the muscles to continue focusing exclusively on the production of ATP while the liver handles the lactate produced in muscle. The cycle also prevents lactate acidosis by removing lactate from the blood. Otherwise, pH would fall as the buffering capacity of blood is exceeded.
Glycogen and marathon running
Since the human body is unable to hold more than approximately 2,000 kcal of glycogen, marathon runners commonly experience a phenomenon referred to as "bonking" or "hitting the wall" around the 20-mile (32-km) point of a marathon. Symptoms of this condition, which signals the depletion of glycogen stores, include general weakness, fatigue, and manifestations of hypoglycemia (low blood sugar), such as dizziness and even hallucinations. This rapid drop in performance results from a shift in the fuel supply: as glycogen stores diminish, ATP must also be generated in part from fatty acid oxidation, which is a slower process than the oxidation of glycogen. The simultaneous utilization of both fuels allows for a balance between endurance and speed, preserving enough glucose to fuel the runner's final push to the finish line.
There are several approaches available to prevent glycogen depletion during a marathon or another endurance exercise such as cycling:
- Carbohydrate loading is used to ensure that the initial glycogen level is maximized. This technique consists of increasing the intake of complex carbohydrates in the final three days preceding the event.
- Consuming food or drink that contains carbohydrates during the exercise will replenish the supply of glucose. This is a requirement for very long distances; it is estimated that Tour de France competitors receive up to 50 percent of their daily caloric intake from on-the-bike supplements.
- Decreasing the intensity of the exercise to the so-called "fat-loss" level (a heart rate of 130 beats per minute for a 30-year-old athlete) will lower both the energy requirements per unit of distance and the fraction of the energy that comes from glycogen.
Disorders of glycogen metabolism
The most common disease involving abnormal glycogen metabolism is diabetes mellitus, which is characterized by persistent variable hyperglycemia (high blood sugar levels), resulting either from a deficiency of insulin or from an inadequate response by the body's cells to insulin. As mentioned above, insulin is the principal control signal for the conversion of glucose to glycogen for storage in liver and muscle cells. Lowered insulin levels result in the reverse conversion of glycogen to glucose by the liver when blood sugar levels fall. With the system out of balance, the liver then releases more glucose into the blood than can be utilized by other cells.
Several inborn errors of metabolism are caused by inherited genetic deficiencies of the enzymes involved in glycogen synthesis or breakdown. Collectively referred to as glycogen storage diseases, they include the following types:
- von Gierke's disease (Type I) is the most common of the glycogen storage diseases. It results from a deficiency of the enzyme glucose-6-phosphatase, which in turn impairs the ability of the liver to produce free glucose from glycogen stores and through gluconeogenesis. Since these are the two primary metabolic mechanisms by which the liver supplies glucose to the rest of the body during periods of fasting, hypoglycemia is symptomatic of the disease. Reduced glycogen breakdown results in increased glycogen storage in liver and kidneys, causing enlargement of both organs. Frequent or continuous feedings of cornstarch or other carbohydrates is the principal treatment.
- Pompe disease (Type II) is caused by a deficiency in a lysosome-specific enzyme of glycogen breakdown called acid alpha-glucosidase (GAA). It is the only glycogen storage disease involving a defect in the lysosome, an organelle that contains digestive enzymes that breakdown macromolecules such as glycogen. The resulting build-up of glycogen causes progressive muscle weakness (myopathy) throughout the body and affects various body tissues, particularly in the heart, skeletal muscles, liver, and nervous system.
- A disorder involving glycogen metabolism in muscle is McArdle's disease (Type V). It is characterized by a deficiency of myophosphorylase, the muscle isoform of the enzyme glycogen phosphorylase. This enzyme participates in the breakdown of glycogen so that it can be utilized within the muscle cell. Persons with this disease experience difficulty when their muscles are called upon to perform relatively brief yet intense activity. The inability to break down glycogen into glucose leads to an energy shortage within the muscle, resulting in muscle pain and cramping, and sometimes causing serious injury to the muscles. In addition, the breakdown of muscle tissue can indirectly lead to kidney damage. Anaerobic exercise must be avoided but gentle aerobic activity is beneficial.
ReferencesISBN links support NWE through referral fees
- Chen Y.-T., and A. Burchell. “Glycogen storage diseases.” In C. R. Scriver, A. L. Beaudet, W. S. Sly, and D. Valle (eds.), The Metabolic Basis of Inherited Disease, 7th edition. New York: McGraw-Hill, 1995.
- Cornblath M, and R. Schwartz. “Disorders of glycogen metabolism.” In M. Cornblath and R. Schwartz, Disorders of Carbohydrate Metabolism in Infancy, 3rd edition. Cambridge: Blackwell, 1991.
- Stryer, L. 1995. Biochemistry, 4th edition. New York: W. H. Freeman.
- Tsalikian E., and M. W. Haymond. “Hypoglycemia in infants and children.” In F. J. Service, Hypoglycemic Disorders: Pathogenesis, Diagnosis, and Treatment. Boston: G. K. Hall Medical Publishers, 1983.
Credits
New World Encyclopedia writers and editors rewrote and completed the Wikipedia article in accordance with New World Encyclopedia standards. This article abides by terms of the Creative Commons CC-by-sa 3.0 License (CC-by-sa), which may be used and disseminated with proper attribution. Credit is due under the terms of this license that can reference both the New World Encyclopedia contributors and the selfless volunteer contributors of the Wikimedia Foundation. To cite this article click here for a list of acceptable citing formats.The history of earlier contributions by wikipedians is accessible to researchers here:
- Glycogen history
- Cori_cycle history
- Glycogen_storage_disease_type_I history
- Glycogen_storage_disease_type_II history
- Glycogen_storage_disease_type_V history
- Bonk_(condition) history
- Glycosidic_bond history
The history of this article since it was imported to New World Encyclopedia:
Note: Some restrictions may apply to use of individual images which are separately licensed.