Cerebrum
Brain: Cerebrum | ||
---|---|---|
The lobes of the cerebral cortex include the frontal (blue), temporal (green), occipital (red), and parietal lobes (yellow). The cerebellum (unlabeled) is not part of the telencephalon. | ||
Diagram depicting the main subdivisions of the embryonic vertebrate brain. | ||
Latin | Cerebrum, telencephalon | |
Gray's | subject #189 817 | |
Artery | anterior cerebral, middle cerebral, posterior cerebral | |
Vein | cerebral veins | |
MeSH | Telencephalon |
The cerebrum is the part of the prosencephalon (forebrain) of the brain that contains the cerebral cortex as well as such subcortical structures as the hippocampus, amygdala, basal ganglia, olfactory bulb, and corpus callosum. In humans, the cerebrum is the superior-most (topmost) region of the central nervous system (CNS). However, in nearly all vertebrates, the cerebrum is the anterior-most region of the CNS as most animals rarely assume an upright anatomical position.
In mammals, cerebrum has been used synonymously with the term "telencephalon," or "end brain"; however, in other vertebrates, the term telencephalon is used to refer to the embryonic structure from which the mature cerebrum develops.
The surface of the cerebral cortex is highly convoluted in large mammals, with the folds and grooves allowing a much greater surface area in a confined space as in the skull. In humans, this highly folded nature with gyri and sulci is particularly pronounced, and allowing some 15–33 billion neurons in the cerebral cortex, each connected by synapses to several thousand other neurons. The cerebrum is also divided into approximately symmetric left and right cerebral hemispheres by a deep grove or fissure.
Latin for "brain," the cerebrum is involved in speech and language, spatial recognition, learning and memory, sensory processing, and olfaction. With the assistance of the cerebellum, the cerebrum controls all voluntary actions in the body. Notably, while the two cerebral hemispheres of humans appear structurally similar, they differ in many functions. For example, in most people, the dominant hemisphere for language is the left hemisphere, where most of the neural processing takes place for speech comprehension, the formation of thoughts into speech, and the generation of motor output for language communication. In most people, the nondominant hemisphere is adept at spatial reasoning and musical ability. Damage to specific areas can cause such conditions as the the inability to recall faces or have fluid and rapid speech but lacking meaning, with the words tossed together.
Overview
All vertebrates and most invertebrates have an enlarged mass of nervous tissue that constitute an organ known as a brain. While sponges lack nervous systems and the nervous system of such radially symmetric symmetric animals as ctenophores (comb jellies) and cnidarians (e.g., anemones, hydras, corals. and jellyfishes) is but a diffuse network of isolated cells (Ruppert et al. 2004), all bilateral animals—with the exception of a few types of worms—have a nervous system containing a brain, a central cord (or two cords running in parallel), and nerves radiating from the brain and central cord. In vertebrates, the brain is the most complex organ in the body. Together the brain and spinal cord of vertebrates comprise what is known as the central nervous system (CNS), with the brain contained in the cranial subcavity (the skull), and the spinal cord in the spinal cavity (within the vertebral column). The human brain itself averages about 86 billion neurons (Gonzalez 2012).
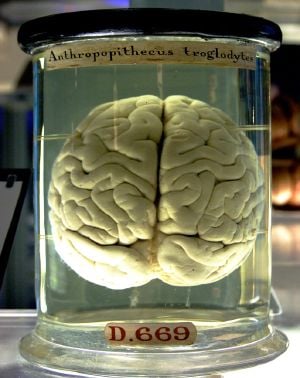
The brains of all species are composed primarily of two broad classes of cells: neurons and glial cells. Glial cells (also known as glia or neuroglia) come in several types, and perform a number of critical functions, including structural support, metabolic support, insulation, and guidance of development. Neurons, however, are usually considered the most important cells in the brain (Kandel et al. 2000). The property that makes neurons unique is their ability to send signals to specific target cells over long distances. They send these signals by means of an axon, which is a thin protoplasmic fiber that extends from the cell body and projects, usually with numerous branches, to other areas, sometimes nearby, sometimes in distant parts of the brain or body. These axons transmit signals in the form of electrochemical pulses called action potentials. Axons transmit signals to other neurons by means of specialized junctions called synapses. A single axon may make as many as several thousand synaptic connections with other cells (Kandel et al. 2000). When an action potential, traveling along an axon, arrives at a synapse, it causes a chemical called a neurotransmitter to be released. The neurotransmitter binds to receptor molecules in the membrane of the target cell (Kandel et al. 2000). Dendrites are another type of protoplasmic extension of the cell body (soma) and these typically receive signals from other neurons and transmit the signals toward the cell body, normally using short-distance graded potentials rather than action potentials (nerve impulses).
Synapses are the key functional elements of the brain (Shepherd 2004). The essential function of the brain is cell-to-cell communication, and synapses are the points at which communication occurs. The human brain has been estimated to contain approximately 100 trillion synapses (Williams and Herrup 1988) and about 97 billion neurons (Gonzalez 2012);
Vertebrate brains are characterized by three primary divisions: hindbrain (or rhombencephalon), midbrain (or mesencephalon), and forebrain (or prosencephalon). The hindbrain includes the medulla oblongata, the pons, and the cerebellum. The hindbrain was the major component of early brains, as seen via casts of fossil agnathans, and remains the major part of fish brains today. The midbrain primarily is composed of the optic tectum, which processes and receives visual information. The midbrain, pons, and medulla also are collectively referred to as the brainstem. The forebrain is dominant in terrestrial vertebrates, where it is the center of the processing sensor information. The forebrain of reptiles, amphibians, birds, and mammals is commonly divided into two regions: the diencephalon, which consists of the hypothalamus and the thalamus, and the telencephalon, or "end brain," which in mammals is called the cerebrum (Raven et al. 2008). The same number of brain divisions are found in most vertebrates, with the notable exception of a lack of a cerebellum in both hagfishes and lampreys (Northcutt 2002).
The telencephalon also is the term used to refer to the embryonic structure from which the mature cerebrum develops. In mammals, the dorsal telencephalon, or pallium, develops into the cerebral cortex, and the ventral telencephalon, or subpallium, becomes the basal ganglia.
If the brain or spinal cord of a vertebrate is extracted and sliced into thin sections, some parts of each section appear dark and other parts lighter in color. The dark parts are known as gray matter and the lighter parts as white matter. White matter gets its light color from the myelin sheaths of axons: the white matter parts of the brain are characterized by a high density of myelinated axons passing through them, and a low density of cell bodies of neurons. Spinal and cerebral white matter do not contain dendrites, which can only be found in gray matter. Gray matter contains dendrites, along with neural cell bodies and shorter, unmylinated axons. Generally, white matter can be understood as the parts of the brain and spinal cord responsible for information transmission (axons); whereas, gray matter is mainly responsible for information processing (neuron bodies). In the human spinal cord, the axons coated with myelin are on the surface and the axon-dendrite networks are on the inside, while in the brain this is reversed (i.e, in the spinal cord, white matter is on the outside, while it is predominately on the inside in the brain (Chamberlin and Narins 2005; Campbell et al. 2008; Marieb and Hoehn 2010).
The cerebrum lies in front or on top of the brainstem and in humans is the largest and best-developed of the major divisions of the brain. The cerebrum is the newest structure in the phylogenetic sense, with mammals having the largest and best-developed among all species. In humans, the cerebrum surrounds older parts of the brain. The limbic, olfactory, and motor systems project fibers from the cerebrum to the brainstem and spinal cord. Cognitive and volitive systems project fibers from the cerebrum to the thalamus and to specific regions of the midbrain. The neural networks of the cerebrum facilitate complex behaviors such as social interactions, thought, judgement, learning, working memory, and in humans, speech and language.
Structure and composition
Several sub-regions are commonly recognized as part of the cerebrum:
- Cerebral cortex, or cortices of the cerebral hemispheres
- Corpus callosum
- Basal ganglia, or basal nuclei
- Hippocampus
- Amygdala
- Olfactory bulb
The cerebral cortex is the outermost layered structure of neural tissue that covers the cerebrum. It is very dense with nerve cells. For mammals, species with larger brains (in absolute terms, not just in relation to body size) tend to have thicker cortices (Nieuwenhuys et al. 1998). The range, however, is not very great—only a factor of 7 between the thickest and thinnest cortices. The smallest mammals, such as shrews, have a neocortical thickness of about 0.5 mm; the ones with the largest brains, such as humans and fin whales, have thicknesses of 2-4 mm.
In large mammals, the surface of the cerebral cortex is highly convoluted, giving a much greater surface area in a confined space as in the skull. A fold or ridge, in the cortex is termed a gyrus (plural gyri) and a groove or fissure is termed a sulcus (plural sulci). This highly folded nature with gyri and sulci is particularly prevalent in humans, resulting in the surface area of the brain ranging from about 1500 to 2000 square centimeters (233 to 465 square inches) (Freudenrich and Boyd 2013). By increasing the surface area, the amount of neurons contained can likewise be increased. In a typical human, the cerebral cortex is estimated to contain 15–33 billion neurons (Pelvig et al. 2008), each connected by synapses to several thousand other neurons.
The cerebral cortex is referred to as gray matter as it consists of cell bodies and capillaries and contrasts with the underlying white matter, that consists mainly of the white myelinated sheaths of neuronal axons. In humans, the cerebral cortex plays a key role in memory, attention, perceptual awareness, thought, language, and consciousness.
The corpus callosum is the structure of white matter fibers that connect the two hemispheres of the cerebrum (see below).
Basal ganglia is the collective term for aggregates of neuron cell bodies and dendrites that produce islands of gray matter within the white matter of the cerebrum.
The amygdala and hippocampus are two of the components of the limbic system (along with the hypothalamus. The limbic system is also known as the "emotional brain," and is an ancient evolutionary structure.
In most vertebrates, the olfactory bulb is the most rostral (forward) part of the cerebrum. In humans, however, the olfactory bulb is on the inferior (bottom) side of the brain.
The cerebrum is divided into two halves by a deep groove (the interhemispheric fissure or medial longitudinal fissure):
- Right hemisphere
- Left hemisphere
These two cerebral hemispheres are connected by the corpus callosum, a bundle of axons that cross the medial longitudinal fissure. The left and right temporal lobes (see below) also communicate by fibers comprising the anterior commissure near the rear of the brain.
The cerebral cortex is further divided typically into four sections or "lobes," with the separation due to several large sulci:
- Frontal lobes
- Parietal lobes
- Occipital lobes
- Temporal lobes
With the division into two hemispheres, this gives a right and left frontal lobe, right and left parietal lobe, right and left occipital lobe, and right and left temporal lobe. These lobes receive their name from the associated overlying bones of the skull: frontal, pariental, occipital, and temporal.
Some recognize two additional lobes: the limbic lobes (containing the limbic system nuclei) and the insular lobes (located internal to the lateral sulcus), although neither are considered true lobes (Dafny 2013).
If not specified, the expression "lobes of the brain" refers to the telencephalon (cerebrum), although the cerebellum also is divided into lobes.
Development
In the developing vertebrate embryo, the neural tube subdivides into four unseparated sections, which develop further into distinct regions of the CNS; these are the prosencephalon, the mesencephalon, the rhombencephalon, and the spinal cord. The prosencephalon develops further into the telencephalon (forebrain or cerebrum), and the much smaller diencephalon. The telencephalon then forms two lateral telencephalic vesicles, which develop into the left and right cerebral hemispheres.
Functions
Language and communication
Speech and language are mainly attributed to parts of the cerebral cortex.
While the two cerebral hemispheres of humans appear structurally similar, they differ in terms of their role in language (as well as in other functions). In ninety percent of right-handed people and about two-thirds of left-handed people, the dominant hemisphere for language is the left hemisphere; that is, most of the neural processing relative to language occurs in the left hemisphere. Within the dominant hemisphere, speech comprehension and the formation of thoughts into speech are attributed to Wernicke's area, while the generation of motor output for language communication is attributed to Broca's area. Wernicke's area is located in the parietal lobe, between the primary visual and auditory areas, and Broca's area is located within the frontal lobe,near the part of the motor cortex responsible for controlling the face. These two regions are interconnected by a large white matter tract, the arcuate fasciculus. Damage to the Broca's area results in expressive aphasia (non-fluent aphasia). Damage to Wernicke's area results in receptive aphasia (also called fluent aphasia), whereby the person's speech may be fluid and rapid but lack meaning, with words tossed together (Raven et al. 2008).
Spatial recognition
The nondominant hemisphere (in most people, this is the right hemisphere) is adept at spatial reasoning (such as drawing a picture or assembling a puzzle), as opposed to the sequential reasoning (such as formulating a sentence) of the dominant hemisphere. The nondominant hemisphere is also associated with musical ability, and damage to the Broca's speech area of the dominant hemisphere may prevent one from speaking but not from singing. Damage to the inferior temporal cortex in the nondominant hemisphere may prevent one from being able to recall faces (prosopagnosia) (Raven et al. 2008).
Learning and memory
Memory in general appears to be dispersed through different brain regions rather than isolated to a particular region. Particular memories have not been tied to particular cortical sites; cortical damage does not appear to selectively remove memories. Memory may be impaired if the temporal lobes are removed, but it is not lost completely and many memories remain in spite of the damage and can be gradually recovered with time (Raven et al. 2008).
Two regions of the temporal lobes, the amygdala and hippocampus, have been shown to be involved in both short-term memory and its incorporation into long-term memory. When these structures are damaged, the processing of recent events into long-term memory is impaired (Raven et al. 2008).
Short-term or working memory also is tied to the dorsolateral prefrontal cortex, an area in the anterior region of the primate brain. The dorsolateral prefrontal cortex is a subcortical region in the brain, in the region called prefrontal cortex.
Explicit or declarative (factual) memory formation is attributed to the hippocampus and associated regions of the medial temporal lobe. This association was originally described after a patient known as HM had both his left and right hippocampus surgically removed to treat severe epilepsy. After surgery, HM had anterograde amnesia, or the inability to form new memories.
Implicit or procedural memory, such as complex motor behaviors, involves the basal ganglia.
Movement
The cerebrum directs the conscious or volitional motor functions of the body. These functions originate within the primary motor cortex and other frontal lobe motor areas where actions are planned. Upper motor neurons in the primary motor cortex send their axons to the brainstem and spinal cord to synapse on the lower motor neurons, which innervate the muscles. Damage to motor areas of cortex can lead to certain types of motor neuron disease. This kind of damage results in loss of muscular power and precision rather than total paralysis.
It functions as the center of sensory perception, memory, thoughts and judgement; the cerebrum also functions as the center of voluntary motor activity.
Sensory processing
The primary sensory areas of the cerebral cortex receive and process visual, auditory, somatosensory, gustatory, and olfactory information. Together with association cortical areas, these brain regions synthesize sensory information into our perceptions of the world around us.
Olfaction
The olfactory bulb in most vertebrates is the most anterior portion of the cerebrum, and makes up a relatively large proportion of the telencephalon. However, in humans, this part of the brain is much smaller, and lies underneath the frontal lobe. The olfactory sensory system is unique in the sense that neurons in the olfactory bulb send their axons directly to the olfactory cortex, rather than to the thalamus first. Damage to the olfactory bulb results in a loss of the sense of smell.
Variation among species
In the most primitive vertebrates, the hagfishes and lampreys, the cerebrum is a relatively simple structure receiving nerve impulses from the olfactory bulb. (The hagfishes and lampreys also are the only vertebrates that appear to be lacking a cerebellum (Northcutt 2002)). In cartilaginous and lobe-finned fishes, and also in amphibians, a more complex structure is present, with the cerebrum being divided into three distinct regions. The lowermost (or ventral) region forms the basal nuclei, and contains fibers connecting the rest of the cerebrum to the thalamus. Above this, and forming the lateral part of the cerebrum, is the paleopallium, while the uppermost (or dorsal) part is referred to as the archipallium. The cerebrum remains largely devoted to olfactory sensation in these animals, despite its much wider range of functions in amniotes (Romer and Parsons 1977).
In ray-finned fishes, the structure is somewhat different. The inner surfaces of the lateral and ventral regions of the cerebrum bulge up into the ventricles; these include both the basal nuclei and the various parts of the pallium, and may be complex in structure, especially in teleosts. The dorsal surface of the cerebrum is membranous, and does not contain any nervous tissue (Romer and Parsons 1977).
In the amniotes, the cerebrum becomes increasingly large and complex. In reptiles, the paleopallium is much larger than in amphibians, and its growth has pushed the basal nuclei into the central regions of the cerebrum. As in the lower vertebrates, the gray matter is generally located beneath the white matter, but in some reptiles, it spreads out to the surface to form a primitive cortex, especially in the anterior part of the brain (Romer and Parsons 1977).<
In mammals, this development proceeds further, so that the cortex covers almost the whole of the cerebral hemispheres, especially in more developed species, such as the primates. The paleopallium is pushed to the ventral surface of the brain, where it becomes the olfactory lobes, while the archipallium becomes rolled over at the medial dorsal edge to form the hippocampus. In placental mammals, a corpus callosum also develops, further connecting the two hemispheres. The complex convolutions of the cerebral surface are also found only in higher mammals (Romer and Parsons 1977). Although some large mammals (such as elephants) have particularly large cerebra, dolphins are the only species (other than humans) to have cerebra accounting for as much as 2 percent of their body weight (Brink 2008).
The cerebrum of birds has evolved along different lines to that of mammals, although they are similarly enlarged, by comparison with reptiles. However, this enlargement is largely due to the basal ganglia, with the other areas remaining relatively primitive in structure. For example, there is no great expansion of the cerebral cortex, as there is in mammals. Instead, an HVC develops just above the basal ganglia, and this appears to be the area of the bird brain most concerned with learning complex tasks (Romer and Parsons 1977).
ReferencesISBN links support NWE through referral fees
- Brink, T. L. 2008. Unit 4: The nervous system. In T. L. Brink, Psychology: A Student Friendly Approach. Retrieved November 16, 2013.
- Campbell, N. A., J. B. Reece, L. A. Urry, et al. 2008. Biology, 8th edition. San Francisco: Pearson/Benjamin Cummings. ISBN 9780805368444.
- Chamberlin, S. L., and B. Narins. 2005. The Gale Encyclopedia of Neurological Disorders. Detroit: Thomson Gale. ISBN 078769150X.
- Dafny, N. 2013. chapter 1: Overview of the Nervous system. Neuroscience Online. Retrieved November 15, 2013.
- Freudenrich, C., and R. Boyd. 2013. How your brain works. How Stuff Works. Retrieved November 16, 2013.
- Gonzalez, R. 2012. The 4 biggest myths about the human brain. 109.com. Retrieved November 12, 2013.
- Kandel, E. R., J. H. Schwartz, and T. M. Jessell. 2000. Principles of Neural Science. New York: McGraw-Hill. ISBN 9780838577011.
- Marieb, E. N. and K. Hoehn. 2010. Human Anatomy & Physiology, 8th edition. Benjamin Cummings. ISBN 9780805395693.
- Nieuwenhuys, R., H. J. Donkelaar, and C. Nicholson. 1998. The Central Nervous System of Vertebrates, vol. 1. Springer. ISBN 9783540560135.
- Northcutt, R. G. 2002. Understanding vertebrate brain evolution. Integ. and Comp. Biol. 42: 743-756.
- Pelvig, D. P., H. Pakkenberg, A. K. Stark, and B. Pakkenberg. 2008. Neocortical glial cell numbers in human brains. Neurobiology of Aging 29(11): 1754–1762. PMID 17544173.
- Raven, P. H., G. B. Johnson, J. B. Losos, K. A. Mason, and S. R. Singer. 2008. Biology, 8th edition. Boston: McGraw Hill. ISBN 9780072965810.
- Romer, A. S., and T. S. Parsons. 1977. The Vertebrate Body. Philadelphia, PA: Holt-Saunders International. ISBN 003910284X.
- Ruppert, E. E., R. S. Fox, and R. D. Barnes. 2004. Invertebrate Zoology, 7 ed. Brooks/Cole. ISBN 0030259827.
- Sakarya, O., K. A. Armstrong, M. Adamska, et al. 2007. A post-synaptic scaffold at the origin of the animal kingdom. PLoS ONE 2(6): e506. PMID 17551586.
- Shepherd, G. M. 2004. The Synaptic Organization of the Brain. Oxford University Press US. ISBN 9780195159561.
- Williams, R. W., and K. Herrup. 1988. The control of neuron number. Annual Review of Neuroscience 11: 423-453. PMID 3284447.
Credits
New World Encyclopedia writers and editors rewrote and completed the Wikipedia article in accordance with New World Encyclopedia standards. This article abides by terms of the Creative Commons CC-by-sa 3.0 License (CC-by-sa), which may be used and disseminated with proper attribution. Credit is due under the terms of this license that can reference both the New World Encyclopedia contributors and the selfless volunteer contributors of the Wikimedia Foundation. To cite this article click here for a list of acceptable citing formats.The history of earlier contributions by wikipedians is accessible to researchers here:
The history of this article since it was imported to New World Encyclopedia:
Note: Some restrictions may apply to use of individual images which are separately licensed.