Difference between revisions of "Hadron" - New World Encyclopedia
Line 11: | Line 11: | ||
According to the [[quark model]],<ref name="Quark model">C. Amsler et al. 2007. [http://pdg.lbl.gov/2008/reviews/quarkmodrpp.pdf Quark Model, in "Review of Particle Physics"]. ''Phys. Lett.'' B667:1. Retrieved September 10, 2008.</ref> the properties of hadrons are primarily determined by their so-called ''valence quarks''. For example, a [[proton]] is composed of two [[up quark]]s (each with [[electric charge]] +2/3) and one [[down quark]] (with electric charge -1/3). Adding these together yields the proton charge of +1. Although the constituent quarks also carry [[color charge]] (nothing to do with visual [[color]]), a property of the strong nuclear force called [[color confinement]] requires that any composite state carry no residual color charge. That is, hadrons must be colorless. There are two ways to accomplish this: three quarks of different colors, or a quark of one color and an [[antimatter|anti-quark]] carrying the corresponding anti-color. Hadrons based on the former are called [[Hadron#Baryons|baryons]] while those based on the latter are called [[Hadron#Mesons|mesons]]. | According to the [[quark model]],<ref name="Quark model">C. Amsler et al. 2007. [http://pdg.lbl.gov/2008/reviews/quarkmodrpp.pdf Quark Model, in "Review of Particle Physics"]. ''Phys. Lett.'' B667:1. Retrieved September 10, 2008.</ref> the properties of hadrons are primarily determined by their so-called ''valence quarks''. For example, a [[proton]] is composed of two [[up quark]]s (each with [[electric charge]] +2/3) and one [[down quark]] (with electric charge -1/3). Adding these together yields the proton charge of +1. Although the constituent quarks also carry [[color charge]] (nothing to do with visual [[color]]), a property of the strong nuclear force called [[color confinement]] requires that any composite state carry no residual color charge. That is, hadrons must be colorless. There are two ways to accomplish this: three quarks of different colors, or a quark of one color and an [[antimatter|anti-quark]] carrying the corresponding anti-color. Hadrons based on the former are called [[Hadron#Baryons|baryons]] while those based on the latter are called [[Hadron#Mesons|mesons]]. | ||
− | Like all [[subatomic particle]]s, hadrons have [[quantum numbers]] corresponding to the [[Representation theory|representations]] of the [[Poincaré group]]: ''J<sup>PC</sup>(m)'', where ''J'' is the [[spin (physics)|spin]], ''P'', the [[parity]], ''C'', the [[C parity]], and ''m'', the [[mass]]. Note that the mass of a hadron has very little to do with the mass of its valence quarks; rather, due to [[mass–energy equivalence]], most of the mass comes from the large amount of energy associated with the strong nuclear force. Hadrons may also carry [[flavour (particle physics)|flavor]] quantum numbers such as [[isospin]] (or [[G parity]]), and [[strangeness]]. All quarks carry an additive, conserved quantum number called [[baryon number]] | + | Like all [[subatomic particle]]s, hadrons have [[quantum numbers]] corresponding to the [[Representation theory|representations]] of the [[Poincaré group]]: ''J<sup>PC</sup>(m)'', where ''J'' is the [[spin (physics)|spin]], ''P'', the [[parity]], ''C'', the [[C parity]], and ''m'', the [[mass]]. Note that the mass of a hadron has very little to do with the mass of its valence quarks; rather, due to [[mass–energy equivalence]], most of the mass comes from the large amount of energy associated with the strong nuclear force. Hadrons may also carry [[flavour (particle physics)|flavor]] quantum numbers such as [[isospin]] (or [[G parity]]), and [[strangeness]]. All quarks carry an additive, conserved quantum number called [[baryon number]] ''(B)'', which is +1/3 for quarks and -1/3 for anti-quarks. This means that baryons have ''B=1'' while mesons have ''B=0''. |
Hadrons have [[excited state]]s known as [[resonance (quantum field theory)|resonances]]. Each ground-state hadron may have several excited states; hundreds of resonances have been observed in particle physics experiments. Resonances decay extremely quickly (within about 10<sup>−24</sup> [[second]]s) via the strong nuclear force. | Hadrons have [[excited state]]s known as [[resonance (quantum field theory)|resonances]]. Each ground-state hadron may have several excited states; hundreds of resonances have been observed in particle physics experiments. Resonances decay extremely quickly (within about 10<sup>−24</sup> [[second]]s) via the strong nuclear force. | ||
− | In other [[phase (matter)|phases]] of [[QCD matter]] the hadrons may disappear. | + | In other [[phase (matter)|phases]] of [[QCD matter]] the hadrons may disappear. For example, at very high temperature and high pressure, unless there are sufficiently many flavors of quarks, the theory of [[quantum chromodynamics]] (QCD) predicts that quarks and gluons will interact weakly and will no longer be confined within hadrons. This property, which is known as [[asymptotic freedom]], has been experimentally confirmed at the energy scales between a [[GeV]] and a [[TeV]]<ref name="Bethke">S. Bethke. 2007. [http://arxiv.org/abs/hep-ex/0606035 Experimental Tests of Asymptotic Freedom]. ''Prog. Part. Nucl. Phys.'' 58:351-386. Retrieved September 10, 2008.</ref>. |
==Baryons== | ==Baryons== | ||
{{main|Baryon}} | {{main|Baryon}} | ||
− | All known baryons are made of three valence quarks, and are therefore [[fermion]]s. They have baryon number ''B=1'', while anti-baryons (composed of three anti-quarks) have ''B=-1''. In principle, some baryons could be composed of further quark-antiquark pairs in addition to the three quarks (or antiquarks) that make up basic baryons. Baryons containing a single additional quark-antiquark pair are called [[pentaquark]]s. Evidence for these states was claimed by several experiments in the early | + | All known baryons are made of three valence quarks, and are therefore [[fermion]]s. They have baryon number ''B=1'', while anti-baryons (composed of three anti-quarks) have ''B=-1''. In principle, some baryons could be composed of further quark-antiquark pairs in addition to the three quarks (or antiquarks) that make up basic baryons. Baryons containing a single additional quark-antiquark pair are called [[pentaquark]]s. Evidence for these states was claimed by several experiments in the early 2000s, but it has since been refuted.<ref name="Pentaquark">C. G. Wohl. 2008. [http://pdg.lbl.gov/2008/reviews/pentaquarks_b801.pdf Pentaquarks, in "Review of Particle Physics"]. ''Phys. Lett.'' B667:1. Retrieved September 10, 2008.</ref> No evidence of baryon states with even more quark-antiquark pairs has been found. |
==Mesons== | ==Mesons== | ||
{{main|Meson}} | {{main|Meson}} | ||
− | + | A meson is a [[boson]] composed of a quark-antiquark pair. It has a baryon number equal to 0 (B = 0). Examples of mesons commonly produced in particle physics experiments include [[pion]]s and [[kaon]]s. The former also play a role in holding atomic nuclei together via the [[residual strong force]]. | |
Hypothetical mesons have more than one quark-antiquark pair; a meson composed of two of these pairs is called a ''[[tetraquark]]''. Currently there is no evidence of their existence. Mesons that lie outside the quark model classification are called [[exotic meson]]s. These include [[glueball]]s (noted below) and hybrid mesons (mesons bound by excited [[gluon]]s). | Hypothetical mesons have more than one quark-antiquark pair; a meson composed of two of these pairs is called a ''[[tetraquark]]''. Currently there is no evidence of their existence. Mesons that lie outside the quark model classification are called [[exotic meson]]s. These include [[glueball]]s (noted below) and hybrid mesons (mesons bound by excited [[gluon]]s). |
Revision as of 00:24, 11 September 2008
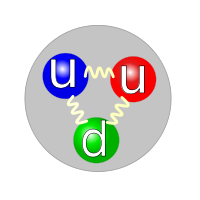
In particle physics, a hadron (from the Greek word ἁδρός, hadros, meaning "thick") is a subatomic particle formed by the binding together of quarks and gluons. The quarks and gluons are held together by what is called the strong interaction or strong nuclear force.[1]
There are two types of hadrons: baryons and mesons. Common examples of baryons are protons and neutrons, which are best-known as constituents of atomic nuclei. Examples of mesons are pions (pi mesons) and kaons (K mesons).
Background
In the 1950s and '60s, scientists discovered many types of subatomic particles that are now classified as hadrons. The concept of quarks was initially formulated to explain these various types of particles.
According to the quark model,[2] the properties of hadrons are primarily determined by their so-called valence quarks. For example, a proton is composed of two up quarks (each with electric charge +2/3) and one down quark (with electric charge -1/3). Adding these together yields the proton charge of +1. Although the constituent quarks also carry color charge (nothing to do with visual color), a property of the strong nuclear force called color confinement requires that any composite state carry no residual color charge. That is, hadrons must be colorless. There are two ways to accomplish this: three quarks of different colors, or a quark of one color and an anti-quark carrying the corresponding anti-color. Hadrons based on the former are called baryons while those based on the latter are called mesons.
Like all subatomic particles, hadrons have quantum numbers corresponding to the representations of the Poincaré group: JPC(m), where J is the spin, P, the parity, C, the C parity, and m, the mass. Note that the mass of a hadron has very little to do with the mass of its valence quarks; rather, due to mass–energy equivalence, most of the mass comes from the large amount of energy associated with the strong nuclear force. Hadrons may also carry flavor quantum numbers such as isospin (or G parity), and strangeness. All quarks carry an additive, conserved quantum number called baryon number (B), which is +1/3 for quarks and -1/3 for anti-quarks. This means that baryons have B=1 while mesons have B=0.
Hadrons have excited states known as resonances. Each ground-state hadron may have several excited states; hundreds of resonances have been observed in particle physics experiments. Resonances decay extremely quickly (within about 10−24 seconds) via the strong nuclear force.
In other phases of QCD matter the hadrons may disappear. For example, at very high temperature and high pressure, unless there are sufficiently many flavors of quarks, the theory of quantum chromodynamics (QCD) predicts that quarks and gluons will interact weakly and will no longer be confined within hadrons. This property, which is known as asymptotic freedom, has been experimentally confirmed at the energy scales between a GeV and a TeV[3].
Baryons
All known baryons are made of three valence quarks, and are therefore fermions. They have baryon number B=1, while anti-baryons (composed of three anti-quarks) have B=-1. In principle, some baryons could be composed of further quark-antiquark pairs in addition to the three quarks (or antiquarks) that make up basic baryons. Baryons containing a single additional quark-antiquark pair are called pentaquarks. Evidence for these states was claimed by several experiments in the early 2000s, but it has since been refuted.[4] No evidence of baryon states with even more quark-antiquark pairs has been found.
Mesons
A meson is a boson composed of a quark-antiquark pair. It has a baryon number equal to 0 (B = 0). Examples of mesons commonly produced in particle physics experiments include pions and kaons. The former also play a role in holding atomic nuclei together via the residual strong force.
Hypothetical mesons have more than one quark-antiquark pair; a meson composed of two of these pairs is called a tetraquark. Currently there is no evidence of their existence. Mesons that lie outside the quark model classification are called exotic mesons. These include glueballs (noted below) and hybrid mesons (mesons bound by excited gluons).
Glueballs
Theoretically, some hadrons can be composed of only gluons, and they have been dubbed glueballs. However, these particles may contain virtual quark-antiquark pairs, at least occasionally, making it difficult to distinguish them from mesons.[1]
See also
Notes
- ↑ 1.0 1.1 Hadrons. Stanford Linear Accelerator Center. Retrieved September 10, 2008.
- ↑ C. Amsler et al. 2007. Quark Model, in "Review of Particle Physics". Phys. Lett. B667:1. Retrieved September 10, 2008.
- ↑ S. Bethke. 2007. Experimental Tests of Asymptotic Freedom. Prog. Part. Nucl. Phys. 58:351-386. Retrieved September 10, 2008.
- ↑ C. G. Wohl. 2008. Pentaquarks, in "Review of Particle Physics". Phys. Lett. B667:1. Retrieved September 10, 2008.
ReferencesISBN links support NWE through referral fees
- Cottingham, W. N., and D. A. Greenwood. 2007. An Introduction to the Standard Model of Particle Physics. 2nd ed. Cambridge, UK: Cambridge University Press. ISBN 978-0521852494.
- Griffiths, David J. 1987. Introduction to Elementary Particles. New York: Wiley. ISBN 0471603864.
- Halzen, Francis, and Alan D. Martin. 1984. Quarks and Leptons: An Introductory Course in Modern Particle Physics. New York: Wiley. ISBN 0471887412.
- Martin, B. R. 2006. Nuclear and Particle Physics: An Introduction. Chichester: John Wiley. ISBN 978-0470025321.
- Povh, Bogdan. 1995. Particles and Nuclei: An Introduction to the Physical Concepts. Berlin: Springer-Verlag. ISBN 0387594396.
- Veltman, Martinus. 2003. Facts and Mysteries in Elementary Particle Physics. River Edge, NJ: World Scientific. ISBN 981238149X.
External links
- Hadrons. Retrieved September 10, 2008.
- Hadrons. Stanford Linear Accelerator Center. Retrieved September 10, 2008.
- Hadrons. The Particle Adventure. Retrieved September 10, 2008.
- The Review of Particle Physics. Particle Data Group. Retrieved September 10, 2008.
Particles in physics | |
---|---|
elementary particles | Elementary fermions: Quarks: u · d · s · c · b · t • Leptons: e · μ · τ · νe · νμ · ντ Elementary bosons: Gauge bosons: γ · g · W± · Z0 • Ghosts |
Composite particles | Hadrons: Baryons(list)/Hyperons/Nucleons: p · n · Δ · Λ · Σ · Ξ · Ω · Ξb • Mesons(list)/Quarkonia: π · K · ρ · J/ψ · Υ Other: Atomic nucleus • Atoms • Molecules • Positronium |
Hypothetical elementary particles | Superpartners: Axino · Dilatino · Chargino · Gluino · Gravitino · Higgsino · Neutralino · Sfermion · Slepton · Squark Other: Axion · Dilaton · Goldstone boson · Graviton · Higgs boson · Tachyon · X · Y · W' · Z' |
Hypothetical composite particles | Exotic hadrons: Exotic baryons: Pentaquark • Exotic mesons: Glueball · Tetraquark Other: Mesonic molecule |
Quasiparticles | Davydov soliton · Exciton · Magnon · Phonon · Plasmon · Polariton · Polaron |
Credits
New World Encyclopedia writers and editors rewrote and completed the Wikipedia article in accordance with New World Encyclopedia standards. This article abides by terms of the Creative Commons CC-by-sa 3.0 License (CC-by-sa), which may be used and disseminated with proper attribution. Credit is due under the terms of this license that can reference both the New World Encyclopedia contributors and the selfless volunteer contributors of the Wikimedia Foundation. To cite this article click here for a list of acceptable citing formats.The history of earlier contributions by wikipedians is accessible to researchers here:
The history of this article since it was imported to New World Encyclopedia:
Note: Some restrictions may apply to use of individual images which are separately licensed.