Nitrogen fixation
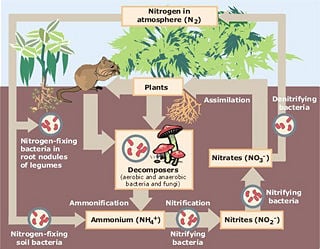
Nitrogen fixation is the process by which nitrogen is taken from its relatively inert molecular form (N2) in the atmosphere and converted into nitrogen compounds useful for other chemical processes (such as, notably, ammonia, nitrate, and nitrogen dioxide). Because atmospheric nitrogen (N2) does not react with other compounds, nitrogen fixation is necessary, as it is an essential nutrient for all living things, including serving as part of nucleic acids and amino acids.
Nitrogen fixation is performed naturally by a number of different prokaryotes, including bacteria. Many higher plants, and some animals (termites), have formed symbiotic associations with these microorganisms. Most species of legumes, for example, provide a habitat for nitrogen-fixing bacteria, and receive a usable form of nitrogen as a benefit.
Human beings have contributed to nitrogen fixation, expressing their creativity by converting N2 to NH3, using fossil fuel as energy. However, the use of such chemically produced nitrogen in fertilizers and munitions have also been expressed in ways that have increased pollution (such as eutrophication of lakes from runoff of excess fertilizer) and war casualties.
Biological Nitrogen Fixation
Biological Nitrogen Fixation (BNF) occurs when atmospheric nitrogen is converted to ammonia by a bacterial enzyme called nitrogenase. Microorganisms that fix nitrogen are called diazotrophs. The formula for BNF is:
- N2 + 8H+ + 8e- + 16 ATP → 2NH3 + H2 + 16ADP + 16 Pi
Although ammonia (NH3) is the direct product of this reaction, it is quickly ionized to ammonium (NH4+). In free-living diazotrophs, the nitrogenase-generated ammonium is assimilated into glutamate through the glutamine synthetase/glutamate synthase pathway. Biological nitrogen fixation was discovered by the Dutch microbiologist Martinus Beijerinck.
Leguminous nitrogen-fixing plants
The best-known plants for fixing nitrogen are legumes (such as clover, beans, alfalfa, and peanuts). These contain symbiotic bacteria called rhizobia within nodules in their root systems, producing nitrogen compounds that help the plant to grow and compete with other plants. When the plant dies, the nitrogen helps to fertilize the soil. The great majority of legumes have this association, but a few genera (for example Styphnolobium) do not.
Crop rotation is often used in agriculture to maintain adequate nitrogen levels in the soil for crop productivity. In this process, leguminous plants, such as alfalfa, are planted in rotation with food crops to replenish nitrogen levels in the soil.
Non-leguminous nitrogen fixing plants
Plants from many other families than legumes have similar associations with nitrogen fixing bacteria, including:
- Lobaria lichen and some other lichens
- Mosquito fern (Azolla species)
- Cycads
- Gunnera
- Alder (Alnus species)
- Ceanothus (Ceanothus species)
- Wax myrtle (Myrica species)
- Mountain-mahogany (Cercocarpus species)
- Bitterbrush (Purshia tridentata)
- Buffalo berry (Shepherdia argentea)
- Ironwood (Casuarina species), Sheoak (Allocasuarina species), and other genera in the Casuarinaceae
Chemical nitrogen fixation
Nitrogen can also be artificially fixed for use in fertilizer, explosives, or in other products. The most popular method is by the Haber process. Artificial fertilizer production has achieved such scale that it is now the largest source of fixed nitrogen in the Earth's ecosystem.
The Haber Process (also Haber-Bosch process) is the reaction of nitrogen and hydrogen to produce ammonia. The nitrogen(N) and hydrogen(H) are reacted over an iron catalyst (Fe) under conditions of 200 atmospheres (atm), 450-500 °C, resulting in a yield of 10-20 percent:
- N2(g) + 3H2(g) ⇌ 2NH3(g) + ΔH ...(1)
(Where ΔH is the heat of reaction or enthalpy. For the Haber process, this is -92.4 kJ/mol at 25 °C)
The process was first patented by Fritz Haber in 1908. In 1910, Carl Bosch, while working for chemical company BASF, successfully commercialized the process and secured further patents. It was first used on an industrial scale by the Germans during World War I: Germany had previously imported "Chilean saltpeter" from Chile, but the demand for munitions and the uncertainty of this supply in the war prompted the adoption of the process. The ammonia produced was oxidized for the production of nitric acid in the Ostwald process, and the nitric acid for the production of various explosive nitro compounds used in munitions.
The Haber process now produces 500 million tons of artificial fertilizer per year, mostly in the form of anhydrous ammonia, ammonium nitrate, and urea. One percent of the world's energy supply is consumed in the manufacturing of that fertilizer (Smith 2002). That fertilizer is responsible for sustaining 40 percent of the Earth's population.
The nitrogen is obtained from the air, and the hydrogen is obtained from water and natural gas in steam reforming:
and shift conversion:
- CO(g) + H2O(g) → CO2(g) + H2(g) ...(3)
Reaction rate and equilibrium
The reaction of nitrogen and hydrogen is reversible, meaning the reaction can proceed in either the forward or the reverse direction depending on conditions. The forward reaction is exothermic, meaning it produces heat and is favored at low temperatures, according to Le Chatelier's Principle. Increasing the temperature tends to drive the reaction in the reverse direction, which is undesirable if the goal is to produce ammonia. However, reducing the temperature reduces the rate of the reaction, which is also undesirable. Therefore, an intermediate temperature high enough to allow the reaction to proceed at a reasonable rate, yet not so high as to drive the reaction in the reverse direction, is required. Usually, 450 °C is used.
High pressures favor the forward reaction because there are 4 moles of reactant for every 2 moles of product, meaning the position of the equilibrium will shift to the right to produce more ammonia. So the only compromise in pressure is the economical situation trying to increase the pressure as much as possible. Usually, 200 atm is used.
The catalyst has no effect on the position of equilibrium; however, it does reduce the activation energy of system and hence in turn increase the reaction rate. This allows the process to be operated at lower temperatures, which as mentioned before favors the forward reaction. The first Haber-Bosch reaction chambers used osmium and uranium catalysts. However, today a much less expensive iron catalyst is used almost exclusively.
The equilibrium constant for this process is given by:
Keq = [NH3]2/[N2][H2]3
As the temperature increases, the concentration of ammonia decreases and hence, in turn, the equilibrium constant decreases. Temperature (°C) : Keq
25: 6.4 x 102
200: 4.4 x 10-1
300: 4.3 x 10-3
400: 1.6 x 10-4
500: 1.5 x 10-5
In industrial practice, the iron catalyst is prepared by exposing a mass of magnetite, an iron oxide, to the hot hydrogen feedstock. This reduces some of the magnetite to metallic iron, removing oxygen in the process. However, the catalyst maintains most of its bulk volume during the reduction, and so the result is a highly porous material whose large surface area aids its effectiveness as a catalyst. Other minor components of the catalyst include calcium and aluminum oxides, which support the porous iron catalyst and help it maintain its surface area over time, and potassium, which increases the electron density of the catalyst and so improves its reactivity.
The ammonia is formed as a gas, but on cooling in the condenser liquefies at the high pressures used, and so is removed as a liquid. Unreacted nitrogen and hydrogen are then fed back in to the reaction.
ReferencesISBN links support NWE through referral fees
- Chapin, F. S. III, P. A. Matson and H. A. Mooney. 2002. Principles of Terrestrial Ecosystem Ecology. New York: Springer. ISBN 0387954430
- Sittig, M. 1979. Fertilizer Industry: Processes, Pollution Control and Energy Conservation. Park Ridge, NJ: Noyes Data Corp. ISBN 0815507348
- Smil, V. 2001. Enriching the Earth: Fritz Haber, Carl Bosch, and the Transformation of World Food Production. Cambridge, MA: MIT Press. ISBN 026219449X
- Smith, B. E. 2002. “Nitrogenase reveals its inner secrets.” Science 297 (5587):1654-1655.
Credits
New World Encyclopedia writers and editors rewrote and completed the Wikipedia article in accordance with New World Encyclopedia standards. This article abides by terms of the Creative Commons CC-by-sa 3.0 License (CC-by-sa), which may be used and disseminated with proper attribution. Credit is due under the terms of this license that can reference both the New World Encyclopedia contributors and the selfless volunteer contributors of the Wikimedia Foundation. To cite this article click here for a list of acceptable citing formats.The history of earlier contributions by wikipedians is accessible to researchers here:
The history of this article since it was imported to New World Encyclopedia:
Note: Some restrictions may apply to use of individual images which are separately licensed.